Abstract
Energy is required for numerous metabolic activities that are necessary to sustain life. The process of respiration enables the body to get oxygen for metabolism as well as to get rid of carbon dioxide, which is the byproduct of metabolism. The lungs are vital organs in these processes. Various experimental procedures can be used to examine the function of lungs in terms of their capacity to take in oxygen and remove carbon dioxide. This experiment aimed at establishing the effect of carbon dioxide on ventilation and the breath-holding capacity in various conditions using a spirometer. It was realized that an increase in carbon dioxide concentration raised the ventilatory response and that an increase in oxygen concentration did not have a significant effect on the ventilatory response. It was also found that intense physical activity reduced an individual’s breath-holding capacity. It was, therefore, concluded that spirometry was an essential procedure in the determination of lung function.
Introduction
Respiration aims to supply tissues with energy and to get rid of carbon dioxide. These aims can be achieved by grouping respiration into four key categories. These are “(1) pulmonary ventilation, which means the inflow and outflow of air between the atmosphere and the lung alveoli; (2) diffusion of oxygen and carbon dioxide between the alveoli and the blood; (3) transport of oxygen and carbon dioxide in the blood and body fluids to and from the body’s tissue cells; and (4) regulation of ventilation and other facets of respiration” (Guyton & Hall 2006, p. 471). Approximately 5% of the entire energy used by the body is required in the course of an ordinary calm respiration for pulmonary ventilation. However, this requirement increases 50 times during intense exercise particularly if the individual has any form of amplified airway resistance. Continued replenishment of gas exchange regions of the lungs that are in proximity to the circulatory system is the ultimate goal of pulmonary circulation. These areas are the alveoli, alveolar sacs and alveolar ducts.
Spirometry, a relatively simple and efficient technique, is used to examine pulmonary ventilation by taking note of the volume of air that moves into and out of the lungs. This technique provides valuable information about the functioning of the lungs during the assessment and diagnosis of respiratory complications such as asthma and chronic obstructive pulmonary disease (Levy et al. 2009). Spirometry is also important in establishing hyperventilation syndrome (Malmberg, Tamminen, & Sovijärvi 2000). A study by Clark et al.(2013) also uses spirometry in the screening of people living around an area with high levels of chlorine exposure in Graniteville, South Carolina.
This experiment aimed at exploring ventilatory changes in response to inspired carbon dioxide. It also intended to measure individual ventilator drive by the establishment of breath-holding capacity.
Materials and Methods
The ventilatory responses were measured by quantifying the alterations in ventilation and breathing frequency when the subject was at rest. That was achieved by letting the subject breathe in known concentrations of carbon dioxide for approximately one minute. Open loop spirometry apparatus were prepared for use after which the gas mixture to be inhaled was also prepared by filling a Douglas bag. The subject was made to sit in such a manner that he was unable to see the experimental results as they were being recorded. The mouthpiece of the spirometer was connected to the subject after which the nose clip was applied. The respondent was allowed two minutes to relax and regularize breathing. The ventilatory response was established by measuring changes in breathing frequency (RR) and ventilation (VE). That was achieved by using a slow scroll velocity of 40 samples per second for 180 seconds. The number of breaths per second was established using different gas mixtures in the Douglas bag. The various gases were medical air, carbogen (5% CO2 and 95% O2), 50% O2 and 50% nitrogen, and 15% O2 and 85% nitrogen. The compositions of gases and the experimental values were recorded in an appropriately labeled table.
To establish the individual respiratory drive, the breath-holding ability was quantified. A one-way valve was fitted to a Douglas bag together with a sterile mouthpiece. The subject was instructed to breathe in fully and then blow progressively. The subject was instructed to hold the last breath until breaking point after he had inhaled some air. The duration within which the breath was held was recorded using a stopwatch. At the breaking point, the exhaled air was forced into the Douglas bag. A sample of the Douglas bag contents was transferred into a re-breathing bag via a small valve by gently pressing the Douglas bag. Subsequently, the sample line from the AD Instrument Gas Analyzer was placed in the open valve on the re-breathing bag sample. This procedure was repeated until there was a steady reading of the lab chart record.
The time taken to hold breath was recorded, and oxygen and carbon dioxide quantities were measured for different air mixtures that were inhaled.
Results
Table 1: Ventilatory responses to carbon dioxide (CO2).
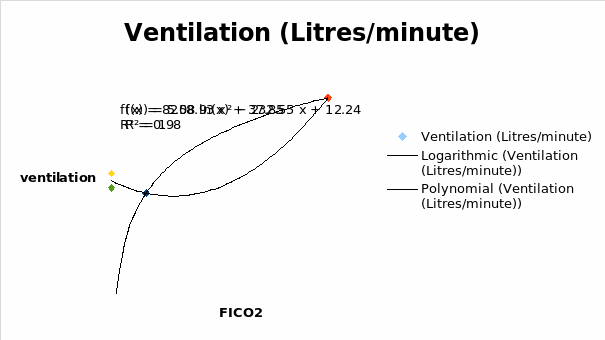
It was seen that the ventilation decreased with an increase in FICO2 until 0.02. From that point, the ventilation increased with an increase in FICO2.
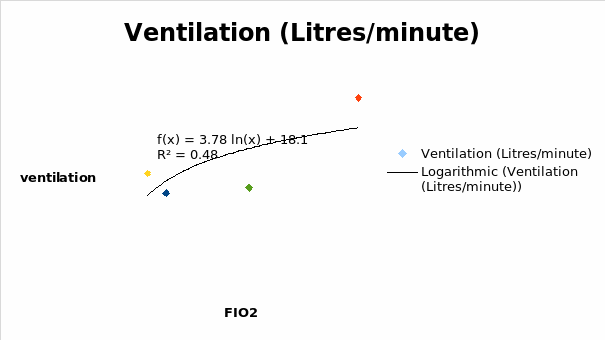
It was realized that ventilation decreased with a decrease in the FIO2 until 0.45. From that point, the ventilation increased reaching a maximum at FIO2 of 1.
Table 2: Breath-holding outcomes.
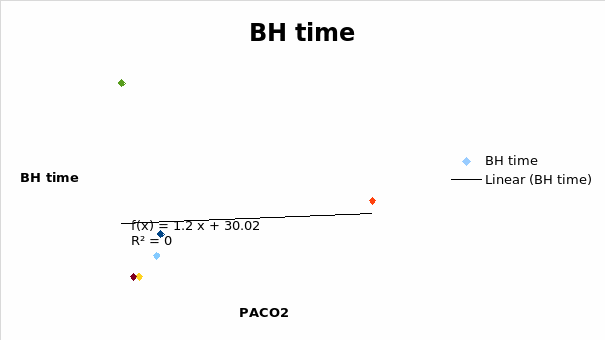
It was seen that the breath-holding capacity increased steadily at PACO2 of approximately 6 and reached the maximum possible at approximately 10 PACO2.
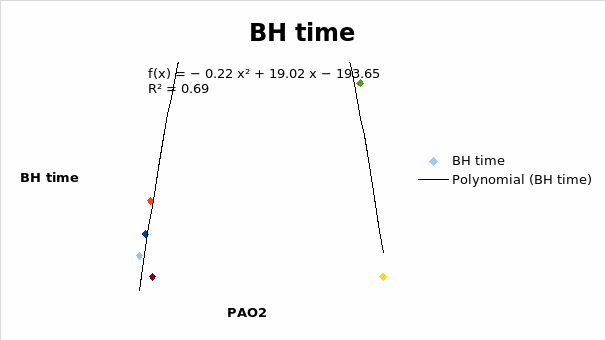
It was seen that the breath holding time increased with an increase in the PAO2 reaching a maximum time of 108 seconds at PAO2 of 45.
Discussion
Lung ventilation increased with an increase in FICO2. The highest ventilatory response was observed at a carbon dioxide concentration of 0.05%. At that concentration, the quantity of carbon dioxide was significantly higher than the normal concentration in air. There was no significant change in ventilation in relation to FIO2. That was because carbon dioxide acted as the chief hypercapnia stimulant (Lindholm & Lundgren 2006) thereby triggering the respiratory center to hyperventilate. When the subject inhaled room air, which comprised 0.008% carbon dioxide, the ventilatory effect was about the normal range. However, increasing the concentration of carbon dioxide in the air raised the ventilation in response to the elevated carbon dioxide. The body, therefore, had to increase its oxygen intake in an attempt to restore equilibrium. At nitrogen concentration of 46.7%, there was no significant increase in ventilation despite there being a high concentration of oxygen (53.3%). However, the ventilatory response was seen to increase slightly at a nitrogen concentration of 86.3%. At that point, the concentration of oxygen in the air was lower than in normal air. According to Guyton and Hall, a reduction in arterial oxygen stimulated the chemoreceptors in the brain leading to increased ventilation (2006). The normal ventilation value from the experiment (10.9 litres per minute) differed slightly from the documented value of between 5 and 8 litres per minute (Guyton & Hall 2006).
One factor that appeared significant in the establishment of ventilatory drive was the concentration of carbon dioxide in the blood. The rate of metabolism played a key role in determining the concentration of carbon dioxide. The quantity of carbon dioxide increased in blood when the overall consumption of oxygen exceeded the lung capacity to drive out the carbon dioxide produced. An increase in carbon dioxide lowered the pH of blood because the gas dissolved to form weak carbonic acid. Therefore, ventilation increased according to the magnitude of carbon dioxide produced when doing exercise. Normally, the carbon dioxide chemoreceptors indicated increasing concentrations of carbon dioxide in the blood leading to ventilation of the lungs. However, some long-term pulmonary ailments could interfere with the functioning of those receptors. Therefore, oxygen concentration became the next crucial determinant of ventilation. Hypoxemia (the reduced oxygen concentration in blood) then drove ventilation.
It was seen that the breath-holding capacity was highest when the percentage oxygen concentration was at its maximum value (100%). The breath-holding capability was lowest during cycling. It was known that while carrying out heavy exercises the rate of oxygen consumption increased tremendously. The level of carbon dioxide produced also increased and caused hypercapnia. The resultant hypercapnia forced one to halt the breath-hold (Lindholm & Lundgren 2006) accounting for the short breath-hold time that was recorded during cycling.
Though the results obtained in the above experiment were rough estimations of the documented literature values, there were slight variations in the normal ventilation values due to experimental errors. It was possible that the instruments introduced some elements of discrepancies in the results.
Another challenge in the use of the spirometry probably arose from disturbances in the subject. Emotions such as tension and anxiety could trigger the release of hormones such as adrenaline (epinephrine), which had an effect on the breathing rate of an individual and the rate of oxygen consumption (Numa, Williams & Dakin 2001). Therefore, it was possible that an anxious or excited subject produced an elevated ventilation rate not only because of the constituents of the inhaled air, but also because of a sudden rush of adrenaline due to excitement. Natural processes such as burping, coughing and straining could also affect ventilation (Guyton & Hall 2006). It was impossible to tell whether the subject was straining or not during the experimental procedure.
According to Gibson, Whitelaw and Niafakas (2002), the normal ranges of the indices used in ventilatory control were extensive and could lead to wrong interpretations of the values hence misdiagnosis when handling patients with respiratory complications. In addition, it was hard to decipher occlusion pressures without further measurements of other control factors in the entire respiratory cycle. Those additional measurements were not always readily available. Breath-to-breath scatter could be present in the data necessitating that the average of several breaths was obtained to come up with accurate results (Gibson, Whitelaw, & Niafakas 2002). In the above experiment, only one subject was used, and it was not possible to obtain as many breaths as was necessary to increase the precision of the results.
Conclusion
From the above experiments and information present in the literature, it was evident that an individual’s oxygen consumption increased with an increase in activity or exercise. Therefore, one of the main restrictions on the amount of exercise that an individual could carry out was the person’s capacity to supply an adequate amount of muscle energy needed for the process of respiration. It was also shown that increasing the concentration of carbon dioxide triggered ventilation. Carrying out such assessments helped determine an individual’s breath-holding capacity as well as the ability to provide the energy required during an exercise. That acted as a valuable tool in deciding the suitability of exercises to individuals.
References
Clark, K. A., Chanda, D., Balte, P., Karmaus, W. J., Cai, B., Vena, J., Lawson, A. B., Mohr, L. C., James J Gibson, J. J., & Svendsen, E. R 2013 “Respiratory symptoms and lung function 8–10 months after community exposure to chlorine gas: a public health intervention and cross-sectional analysis,” BMC Public Health, vol.13 no.945, pp. 1-11.
Gibson, G. J., Whitelaw, W., & Niafakas, S 2002, “Tests of overall respiratory function,” American Journal of Respiratory and Critical Care Medicine, vol.166. no. 2002, pp 518–624.
Guyton, A. C. & Hall, J. E 2006, Textbook of medical physiology, 11 edn, Elsevier Saunders, Philadelphia, Pennsylvania.
Levy, L. M., Quanjer, P. H., Booker, R., Cooper, B. G., Holmes, S., & Small, R 2009, “Diagnostic spirometry in primary care: proposed standards for general practice compliant with American Thoracic Society and European Respiratory Society recommendations,” Primary Care Respiratory Journal, vol.18 no.3, pp. 130-147.
Lindholm, P. & Lundgren, C. E. G 2006, “Alveolar gas composition before and after maximal breath-holds in competitive divers,” UHM, vol. 33 no. 6, pp. 463-467.
Malmberg, P. L., Tamminen, K., & Sovijärvi, A. R. A 2000, “Orthostatic increase of respiratory gas exchange in hyperventilation syndrome,” Thorax, vol.2000 no.55, pp. 295–301.
Numa, A. H., Williams, D. G., & Dakin, C. J 2001, “The effect of nebulized epinephrine on respiratory mechanics and gas exchange in bronchiolitis,” American Journal of Respiratory and Critical Care Medicine, vol.164 no. 1, pp.86-91.