Introduction
Glutathione is a unique peptide found in the cells of all eukaryotic organisms. Unlike other peptides formed by matrix synthesis or posttranslational modification, it has its own metabolic pathway. This compound plays a crucial role in cellular metabolism, participating in the maintenance of redox potential and in the detoxification processes of xenobiotics of endo- and exogenous origin, both directly and as a substrate for a number of biotransformation enzymes (Erkekoglu, 2018). Age-related changes, stimulation of immune reactions, and development of diverse diseases are associated with glutathione synthesis. In particular, almost all major human diseases are accompanied by the variability of glutathione levels and oxidative status in cells (Wade & Simek, 2022).
The evolution of life on Earth since the appearance of oxygen in the atmosphere has been accompanied by the development of a biochemical system of antioxidant protection in cells. One of its most important components is reduced glutathione (GSH), a tripeptide L-γ-glutamyl-L-cysteinyl glycine (Erkekoglu, 2018). The small size of the molecule and a sulfhydryl group in the cysteine side chain make glutathione a universal participant in the vast majority of reactions to prevent the damaging effects of reactive oxygen species (ROS) as well as free-radical processes (Erkekoglu, 2018).
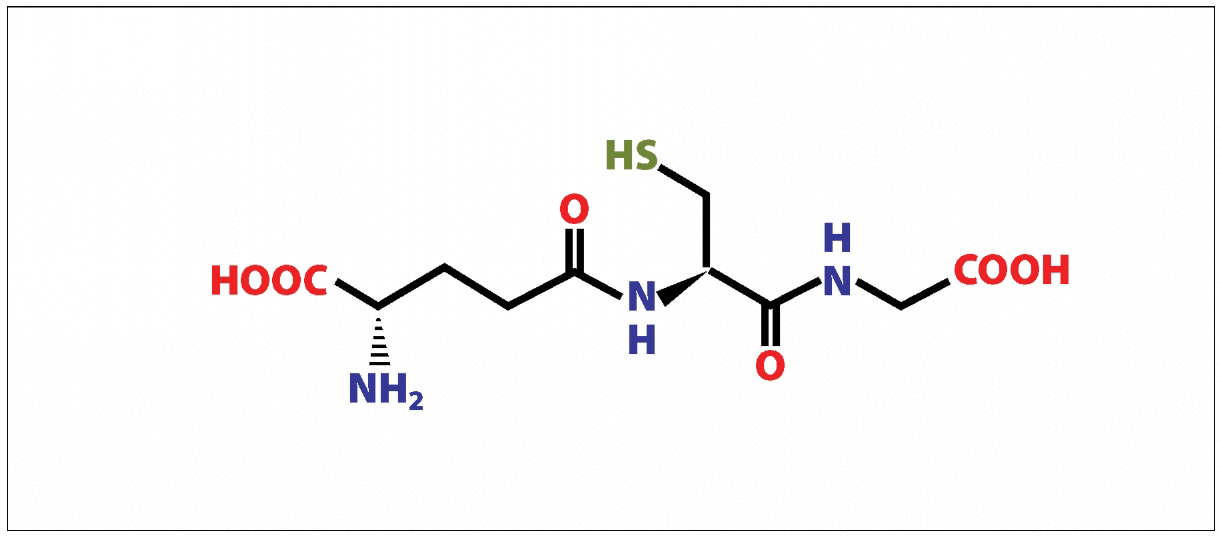
Glutathione, being a peptide in the classical sense, is nevertheless a non-protein tripeptide; it is not formed by matrix synthesis or posttranslational modification. The de novo synthesis of glutathione takes place essentially in the cytosol in two steps, catalyzed by glutamate-cysteine ligase and glutathione synthase. The peculiarity of the first stage of synthesis is the creation of a peptide bond by joining not the α- but the γ-carboxyl group of glutamic acid to the amino group of cysteine (Brown et al., 2022). Because of this unusual structure of the molecule, hydrolysis of such a peptide bond is performed by a single membrane enzyme, γ-glutamyltranspeptidase, located only on the outer surfaces of specific cell types. Due to this, GSH is unyielding to intracellular degradation and is metabolized extracellularly only in tissues with γ-glutamyltranspeptidase. GSH metabolism is very fast; for example, in a rat liver, its half-life period is only 2-3 hours (Erkekoglu, 2018). For mammals, glutathione homeostasis in cells is maintained by three mechanisms (Clarke, 2017). These are de novo synthesis, transport of exogenous GSH through plasma membranes, and extraction from the oxidized form (Clarke, 2017).
De Novo Synthesis
De novo synthesis of GSH proceeds in two ATP-dependent steps, which are included in a cycle of six enzymatic reactions called the γ-glutamic cycle. The first stage is the reaction of peptide bond formation between cysteine and glutamic acid, which is catalyzed by γ-glutamylcysteinligase (γ-GCL) and is the rate-limiting reaction in GSH synthesis. The second stage is a reaction catalyzed by glutathione synthetase (GS), which leads to the creation of GSH as a result of glycine binding to γ-glutamylcysteine (Clarke, 2017). The enzyme capable of hydrolyzing the specific bond in the GSH molecule between glutamic acid and cysteine residues, g-glutamyltransferase (γ-GT), is localized on the outer side of the cytoplasmic membrane of specific cell types and ensures the transfer of the γ-glutamic residue to the amino acid, making its transport into the cell possible (Brown et al., 2022). The cysteinyl glycine dipeptide produced by γ-GT is cleaved by dipeptidase to form cysteine and glycine, which become substrates for γ-GCL and GS, respectively. γ-Glutamylcyclotransferase ensures that the bond of the γ-glutamyl residue with the amino acid is broken to form a free amino acid and 5-oxoproline, which is decyclized by oxoprolinase to form glutamic acid, which also becomes a substrate for γ-GCL.
Thus, extracellular glutathione can be broken down; its amino acids are able to get inside the cell again, where they can be incorporated into the GSH molecule again. Most of the blood plasma GSH content is provided by its synthesis in the liver, so failures of this process in this organ lead to systemic interorgan disorders of glutathione homeostasis (Flohé, 2019). GSH content is replenished not only by de novo synthesis but by the activity of glutathione reductase (GR), which restores GSSG to GSH as well (Flohé, 2019).
Role of Glutathione in Detoxification
The most important function of glutathione is its participation in the processes of detoxification of xenobiotics. These compounds are electrophiles and form conjugates with GSH both spontaneously and enzymatically, in reactions catalyzed by glutathione S-transferase (Dasari et al., 2018). The resulting conjugates are usually excreted from cells, for example, from hepatocytes into bile. In addition, they can undergo cleavage catalyzed by γ-glutamyltranspeptidase, with the formationof a residue of γ-glutamic acid and cysteinyl glycine conjugate (Dasari et al., 2018). The connection between cystine and glycine is broken by dipeptidase, and, as a result, a xenobiotic conjugate with cysteine is formed. This is followed by N-acetylation of this compound to form mercapturic acid (Dasari et al., 2018). The process of detoxifixation and the role of glutathione in the process is shown in Figure 2.
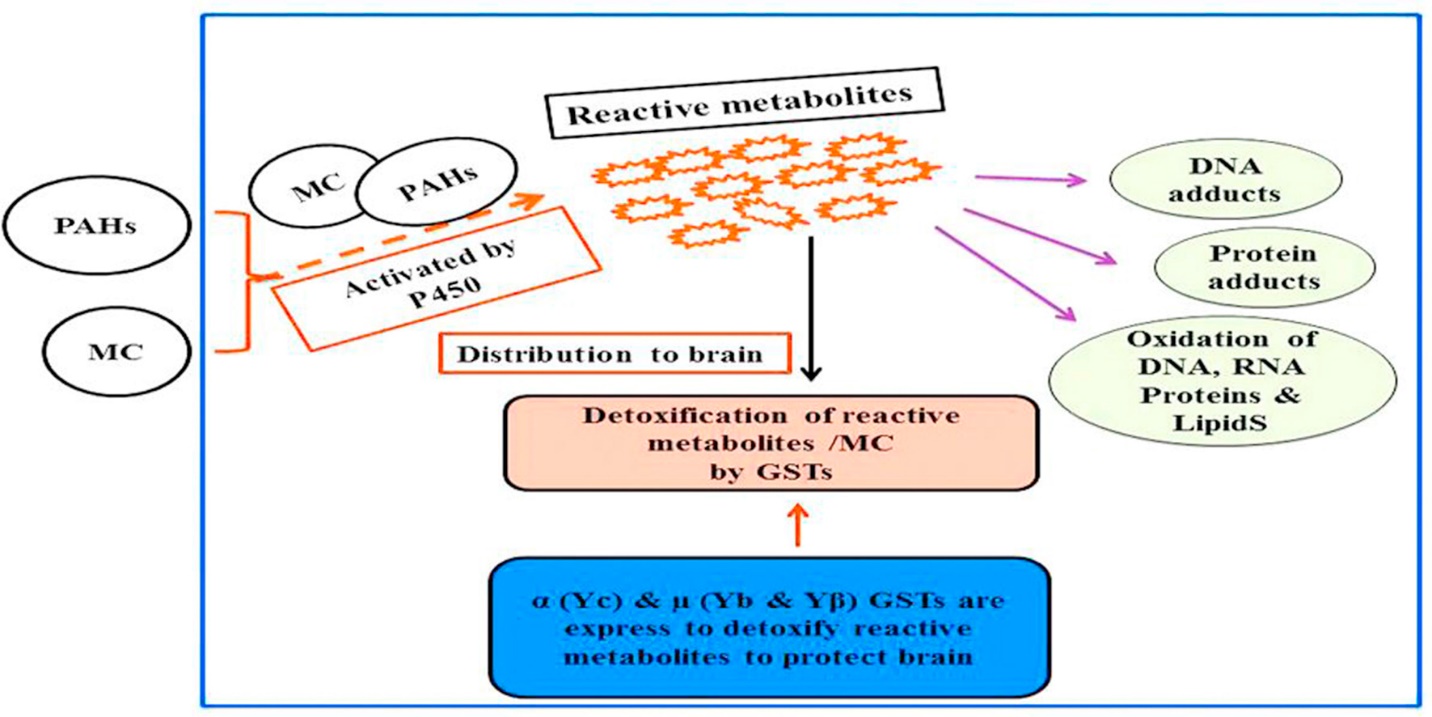
The metabolism of GSH conjugates to mercapturic acid begins in the bile ducts, intestines or kidneys, and the formation of N-acetylcysteine conjugates usually occurs in the kidneys. Similarly, toxic products of endogenous origin are metabolized. Although most conjugation reactions lead to detoxification, it happens that the resulting product remains highly active. Intracellular glutathione is irreversibly consumed during conjugation with xenobiotics. A high level of oxidative stress can exceed the cell’s capacity to transform GSSG into GSH, which leads to the accumulation of GSSG (Dasari et al., 2018). To protect the cell from a shift in the redox equilibrium, GSSG can be actively exported from cells or react with sulfhydryl groups of proteins, which leads to the formation of mixed disulfides that deplete cellular GSH reserves (Dasari et al., 2018).
In cells such as lymphocytes and fibroblasts, an increased level of GSH is associated with an early proliferative response and is important for cells entering the S-phase. In proliferating primary cultures of low-density hepatocytes, an increase in GSH concentration stimulated a G0 phase shift before the G1 phase of the cell cycle. It is known that GSH modulates DNA synthesis by maintaining the level of reduced glutaredoxin or thioredoxin, which are necessary for the activation of ribonucleotide reductase, an enzyme that limits the rate of DNA synthesis (Dasari et al., 2018).
Glutathione is involved in the implementation of cell death processes. One of the final stages of life, apoptosis, is characterized by such morphological features as chromatin condensation, and fragmentation. GSH regulates the redox status of specific thiol proteins, such as stress kinases and caspases involved in cell death. During apoptosis, the level of GSH decreases in mammilian cells, the export of GSH from cells increases, the activity of γ-glutamylcysteinyl ligase decreases (Scirè et al., 2019).
With a very high leakage of GSH, cell death induced by various agents passes from the stage of apoptosis to the stage of necrosis. This means that high levels of ROS can suppress the mechanism of apoptosis. It has been shown that in rabbits, with age, there is a decrease in the concentration of glutathione in all tissues due to gene expression γ-glutamylcysteinyl ligases and glutathione-tetases (Scirè et al., 2019). Similar results were obtained on the role of glutathione in the functioning of antioxidant defense and biotransformation systems in mice. The conducted studies indicate the probability of a link between a decrease in the concentration of GSH and many age-related diseases. Nevertheless, the influence of age on the expression of γ-glutamylcysteinyl ligase and glutathione synthetase remains largely unexplained (Scirè et al., 2019).
Glutathione Transferases
The living cell uses three lines of enzymatic defense against active oxygen compounds with superoxide dismutase, catalase, and glutathione peroxidase; glutathione peroxidase and glutathione transferase. These three lines of defense sequentially reduce superoxide radicals, H2O2, and organic hydroperoxides (Brown et al., 2022). There is also a fourth line of defense, the neutralization of secondary products of reoxidation of other oxidized compounds, in which glutathione transferase, glyoxylate, and formaldehyde dehydrogenase are involved. Glutathione participates in three of the four defense lines and, therefore, makes the main contribution to the process effectiveness (Erkekoglu, 2018).
Glutathione, glutathione peroxidase, glutathione transferase, glutathione reductase, and NADP-H form the glutathione antioxidant system, in which NADP-H glutathione reductase is essential to reduce oxidized glutathione and recycle it. Reduction by glutathione peroxidase and glutathione hydroperoxide transferase prevents the progression of peroxidation and the appearance of its secondary metabolites (Solomon et al., 2019). Glutathione transferases play an essential role in the neutralization of secondary peroxidation products and other oxidized substances (Daniels, 2019). They conjugate with glutathione the main and most toxic product of lipid peroxidation (Campbell, 2017). Organic peroxides can be reduced by glutathione peroxidase and glutathione S-transferase; hydrogen peroxide can also be reduced by catalase, which can be found only in peroxisomes (Overby & Chang, 2022). Since there is no catalase in mitochondria, the importance of GSH to mitochondria is undeniable. Therefore, mitochondrial GSH levels are critical to combat oxidative stress, both physiological and that arising from pathological conditions (Brown et al., 2022).
Glutathione’s Role in Oxidative Stress
High levels of oxidative stress can exceed the cell’s ability to restore GSSG to GSH, which leads to an accumulation of GSSG. GSSG can be actively exported from cells or react with sulfhydryl groups of proteins, resulting in the creation of mixed disulfides that deplete cellular GSH stores (Campbell, 2017). In lymphocytes and fibroblasts, elevated GSH levels are associated with proliferative response and are important for cells entering the S-phase. In proliferating primary cultures of low-density rat hepatocytes, increased GSH concentration stimulated a shift of the G0 phase to the G1 phase of the cell cycle. Partial hepatectomy was found to produce a similar effect (Campbell, 2017).
If the GSH level increase is blocked after this surgery, liver regeneration slows down. GSH level directly correlates with the growth of liver carcinoma cells. It has been shown that an increase in GSH concentration promotes the growth of melanoma metastases in the liver. However, the peculiarities of the molecular mechanism of cell proliferation modulation by GSH have not been elucidated to a full extent. It is known that GSH modulates DNA synthesis through maintaining the level of reduced glutaredoxin or thioredoxin necessary for the activation of ribonucleotide reductase, the rate-limiting enzyme of DNA synthesis (Wade & Simek, 2022).
Conclusion
Thus, glutathione is an essential component of the cell’s antioxidant system. In addition, it is involved in disulfide bond formation, xenobiotic neutralization, and metabolite transport and is part of several cell signaling pathways. Glutathione synthesis is brought about by enzymes localized in the cytosol, γ-glutamatecysteinligase, and glutathione synthetase. The glutathione anti-peroxidase system effectively protects cells from oxidative stress, and usually, only when it is deficient or depleted do serious lesions occur. In terms of the danger of developing a number of chronic non-infectious diseases grouped in the group of free-radical pathology. Modern scientists should strive to avoid not only the depletion of glutathione but the whole pool of antioxidants that function as part of the physiological antioxidant system of the mammalian organism. There is significant necessity in further research on the mechanisms of antioxidant functioning of glutathione in different organs.
Reference List
Brown, W.H., Iverson, B.L. and Anslyn, E.V. (2022) Organic Chemistry. Pacific Grove: Brooks.
Campbell, N.A. (2017) Biology. Upper Saddle River: Pearson.
Clarke, G. (2017) Zoology. Manchester: Carcanet.
Daniels, P. (2019) Mammals. Washington, D.C.: National Geographic.
Dasari, S., Ganjayi, M. S., Yellanurkonda, P., Basha, S., & Meriga, B. (2018). Role of glutathione S-transferases in detoxification of a polycyclic aromatic hydrocarbon, methylcholanthrene. Chemico-biological interactions, 294, 81-90. Web.
Erkekoglu, P. (2018) “Introductory chapter: A general overview of glutathione, Glutathione Transport, and glutathione applications,” Glutathione in Health and Disease. Web.
Flohé L. (2019) Glutathione. Boca Raton: CRC Press.
Overby, J. and Chang, R. (2022) Chemistry. New York: McGraw Hill.
Scirè, A., Cianfruglia, L., Minnelli, C., Bartolini, D., Torquato, P., Principato, G.,… & Solomon, E.P. et al. (2019) Biology. Boston, MA, USA: Cengage.
Wade, L.G. and Simek, J.W. (2022) Organic Chemistry. Upper Saddle River: Pearson