Introduction
In the past few decades, Green Fluorescent Protein (GFP) has gained popularity in the fields of cellular biology, biotechnology and microscopy (Chalfie & Kain 2005). The GFP is made up of a total of 238 amino acid residues. These amino acid residues are responsible for the green fluorescence that is a characteristic of the GFP under UV light. GFP has the capacity to transfer energy and through this process, the protein transduces the blue chemiluminescence of aequorin, another type of protein, into green fluorescent light (Snapp 2009). GFP was first discovered by Shimomura and colleagues in 1962. Since then, GFP has continued to attract significant interest from various scientists. The protein is now among the most exploited and studied proteins in biology and biochemistry (Tsien 1998). Its phenomenal capability to produce a noticeable fluorosphore, coupled with its highly organized structure ensures that GFP remains an ideal protein model for study. With time, more universities and other institutions of higher learning will continue to rely on GFP as a standard teaching tool in the field of molecular biology and biochemistry (Tsien 1998). The current essay is an attempt to examine the applications of Green Fluorescent Protein (GFP) in cell biology, biochemistry, and biotechnology. To do so, the essay shall endeavor to outline the sources of GFP. In addition, its structural features shall also be explored, along with how this has played a role in its usefulness. Moreover, the general strategies that are essential for the application of GFP in cell biology, biochemistry and biology shall also be examined. In addition, the essay shall also attempt to explore two experimental studies where GFP has been applied.
Sources of GFP
GFP was first isolated from the jellyfish Aequoria aequoria by Shimomura and colleagues in 1962. While undertaking the isolation, the authors noted in their study that one of the distinctive properties of the Aequoria aequoria is that it had the ability to emit a greening luminescence. While carrying out the isolation, Shimomura et al (1962) also observed that the aequoria emitted blue luminescence, as opposed to the green luminescence that often characterizes the intact organism. Once the authors had made detailed observation of the green luminescence, the protein was thereafter purified characterized, and assigned the name Green Fluorescent Protein (GFP).
Most bioluminescent coelenterates in the classes of anthrioza and hydrozoa contain high levels of Green Fluorescent Proteins. It is yet to be clarified whether GFPs occur in the phylum ctenophore and among the scyphozoans. Naturally occurring GFPs emit light of between 490 and 520 nm (Misteli & Spector 1997). The hydrozoan jellyfish is the only known naturally occurring GFP molecule and has an excitation maximum in the UV (Ultraviolet) region of 395 nm. Traditionally, GFP is used in reference to the protein that Shimomura et al (1962) first isolated from Aequorea Victoria. However, other marine organisms have also been shown to possess the same green fluorescent proteins. Since the characterization of Aequores GFP, scientists have continued with their work on other types of GFPs, leading to the characterizations of additional GFPs like Renilla. The basic chromophore features of both Aequorea and Renilla GFP are almost identical, the only difference being that Renilla GFP has a much higher extinction coefficient in comparison with that of Aequorea GFP. Moreover, Renilla GFP has been shown to be resistant to PH-induced denaturation and conformational changes (Harpur, Wouters & Bastiaens 2001), not to mention that it also has the tendency to dimerize.
Physical and Spectral Properties of GFP
GFP has a documented absorbance peak at 295 nm. However, the average emission peak of FP is 508 nm. As the excitation continues the 395 nm peak declines with time (Zimmer 2002). GFP typical GFP structure consists of a single β-sheet that is covalently bonded to the HBI chromophore. The β-sheet also runs right through the center of the GFP (Zimmer 2002). HBI is not able to fluorescence when the GFP scaffold is missing. The GFP barrel consists of inward-facing side chains whose role is to induce certain cyclinization reactions. This in turn induces HBI ionization to the chromophore and phenolate in a process referred to as maturation. The interaction of the GFP barrel side chains with hydrogen bonding and electron-stacking has a huge effect on the color and intensity of GFP. In addition, it also affects the derivatives of GFP in the same way. The GFP barrel is normally tightly-packed, effectively excluding solvent molecules. This is important for the protection of the chromophore fluorescence against quenching by water.
Single GFP molecules are reported to display off/on switching and blinking when observed photochemically. Once the GFP has emitted a given number of photons, it tends to switch off to what is known as a dark state, lasting for approximately 5 minutes. It is important to note that the dark state differs from the off-blinking behavior (Dickson et al 1997). According to Garcia-Parajo (2000), blinking is largely photo-induced. Separately, Dickson et al (1997) suggest that a reversible changeover between a dark transition of an unknown identify and the emitting anionic form could be the reason behind this behavior.
Fluorosphore
In GFP, an internal Ser-Tyl-Gly sequence acts as the originator of fluorosphore. As such, the fluorosphore is made up of Ser65-dehydroThy66-Gly67 residues of the GFP protein. The backbone of these three residues is cyclic in nature and it forms an imidazolidone ring (Zimmer 2002). However, the Ser-Tyr-Gly tripeptide does not have fluorescence, and this is indicative of the fact that the GFP fluorescence is very unique. The fluorosphore comes about as a result of an autocatalytic process, meaning that the sequential mechanism does not require any enzymatic reactions or cofactors. Gly 67 and Ser 65 form a rapid cyclization thus initiating an auto-catalytic process (Creemers et al 2000). Next, the Tyr 66 side chain is oxidized leading to the formation of the fluorosphore. This is a thermosensitive reaction. There is a decline in the amount of fluorosphore formed. On the other hand, GFP is largely stable once formed.
GFP structure
A lot of effort has been dedicated to studies involving the chromosphores of GFP-like proteins. These studies are useful in that they have helped to shed light on the chemical structure of chromophores. In addition, the studies have also helped to shed light on how the GFP molecule is formed. One of the defining characteristics of the GFP structure is its cylindrical fold made up of two protomers (Gerdes & Kaether 1998). By studying the crystal structure of GFP, scientists have revealed that the ability of the chromophore to fluorescence is the main reason why GFP has found wide application in various fields.
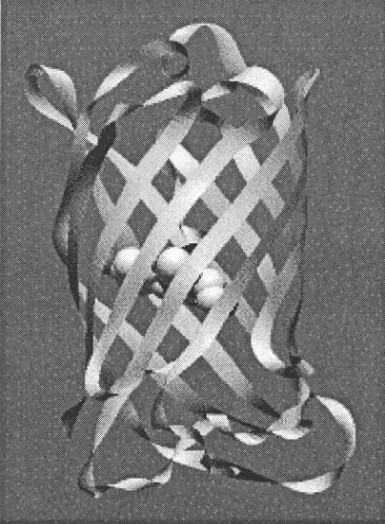
An 11 stranded β-barrel is involved in the formation of GFP. This strand folds into a β-can structure (Zimmer 2002), thereby completely burying the chromophore into the epicenter of the β-can structure. The β-can structure helps to protect the chromophore against the effects of solvent-quenching. Other proteins cannot also reach the chromophore region of the GFP protein since it is folded into the β-can structure. Therefore, the spontaneous formation of chromophore requires a nonenzymatic mechanism. It would also be very hard to truncate GFP to also molecular weight owing to the β-can structure (Shaner, Patterson & Davidson 2007). Nearly the whole of the GFP sequence takes part in the creation of the β-can structure. As such, if any part of the sequence is to be deleted, the entire conformation would be disrupted.
Moreover, multiple covalent interactions help to stabilize the barrel of the GFP.
Owing to this stability, the GFP demonstrates high stability to chemical and thermal denaturation (Shaner et al 2007). The GFP also tends to be quite resistant to proteolysis. Other GFP- like proteins also demonstrates a tendency to oligomerize.
GFP Application in Cell Biology
GFP has found wide application in cell biology as a biological marker following the discovery that its fluorescence does not require a cofactor. In addition, the use of GFP does not change the normal localization or function of the enjoined partner. Also, in order to localize GFP, it is not necessary to undertake substrate entry and fixation permeabilization (Day 1998). Consequently, organelles, cells and proteins that have been marked with GFP can easily be examined in living situations. This breakthrough has enabled biologists to investigate the developmental processes and cellular dynamics in intact cells. Other than the broad impact that GFP technology has had on basic research, the protein has also found use in such areas as assessment of viral vector in human genome therapy (Bastiaens & Pepperkok 2000). GFP is also increasingly being used in monitoring the activity of genetically altered microbes, high throughput drug screening and biological pest control. More importantly, GFP technology has found application in the discovery of drugs.
Some of the properties of GFP that renders it applicable in drug discovery include ease of use, real-time kinetics, and cost savings (Chalfie et al 1994; Chudakov et al 2010). These properties have seen GFP replace other markers like β-galactose and luciferase. GFP fluorescence has also found wide application in determining a significant collection of behaviors and properties. This is mainly the case because the production process of the chromophore of GFP occurs via an internal post-translational autocatalytic cyclization. This cyclization does not need any substrates of cofactors. In addition, the protein’s mobility or activity is rarely affected by the fusion of GFP to a protein (Wouters, Verveer & Bastiaens 2001). Also, GFP is largely nontoxic, not to mention that it is also reported to show resistance to alkaline PHJ, photobleaching, heat, detergents, organic salts, chaotropic salts, and a host of proteases.
Reviews of GFP application in microscopy, biotechnology and cell biology GFP and as a reporter gene have been published. A GFP gene can be used to monitor gene expression by measuring the GFP fluorescence. In this case, GFP fluorescence acts as a direct indicator of the level of gene expression in tissue. Although GFP has been widely used as a reporter gene, on the other hand, the lack of signal amplification means that it has low sensitivity (Zimmer 2002). This is because there is only one chromophore for each GFP, and this tends to limit its use.
GFP has also been used as fusion tags. In this case, the GFP is fused with a cloned gene using standard subcloning methods. The ensuing chimera is expressed in an organism or cell (Bastiaens & Pepperkok 2000). Such GFP fusion tags are very useful in monitoring protein localization, in addition to visualizing dynamic cellular events. Since GFP does not need any substrates or cofactors, this makes it an ideal fluorescence fusion protein marker. The production of the chromophore takes place in vivo, while the ensuing chimera rarely affects the activity or localization of tagged proteins. Consequently, GFP fusion proteins have found wide application in the field of biotechnology.
GFP has found valuable application as a biological marker since its fluorescence does not require any additional cofactors. When the peptide backbone of the molecule crystallizes the fluorosphore is formed (Zimmer 2002). This enables scientists to locate the position of proteins in cells. In addition, Zimmer (2002) reports that the reason why scientists prefer using GFP as a tag is that it does not interfere with the localization of the fusing partner. GFP is also actively used as a cell marker. Other scientists report of its use as an active indicator of calcium sensitivity. Besides, GFP plays an important role in transcription factor dimerization (Bastiaens & Pepperkok 2000), as well as protease action. Recently, biochemical engineers have also discovered that GFP has the potential to quantitatively assess gene expression in various organisms.
Modified forms of GFP have also found use in the making of biosensors. Scientists have managed to introduce the GFP gene into organisms and at the same time, maintain it in their genome through vector injection, bleeding, or cell transformation (Pepperkok et al 1999). GFP is also increasingly being used in FRE (fluorescence resonance energy transfer) applications (Bastiaens & Pepperkok 2000; Wouters, Verveer & Bastiaens 2001). The reason why some of the spectral variants of GFP are seldom utilized as FRET partners is that the donor-acceptor emission spectra are not entirely separated, while the chromophore is located at the very core of the GFP. Also, some of the spectral variants of GFP tend to fluorescence at comparatively low intensities. FRET was first utilized as a calcium probe (Miyawaki et al 1999). In this case, FRET has found use in the determination of calcium concentrations. Day (1998) also reports that FRET has been quite valuable in studies involving protein-protein interactions. Separately, Harper (2001) has published a paper on FRET imaging technique capable of getting around the need for two spectrally unique FRET partners. In this study, Harper (2001) reports on the use of fluorescent lifetime imaging microscopy (FLIP) as a technique for evaluating the fluorescence lifetime released by an EGFP/EYFP pair that was earlier unusable and spectrally similar for FRET. Creemers et al (2000) also report that GFP has found application in high-resolution, low-temperature spectroscopy.
Experimental studies involving GFP
Ohashi et al (2007) conducted an experimental study of GFP-based fluorescence resonance energy transfer (FRET). In their experiment, the authors inserted intrinsically or folded unstructured proteins between Ypet and Cypet. In their experimental study, Ohashi et al (2007) also revealed that enhanced dimerization of GFP molecules led to improved FRET signal. In addition, the researchers recorded a moderate FRET signal with single a fibronectin insert, but no FRET signal was recorded with the double fibronectin. Based on their experimental study, the authors concluded that GFP-based FRET might prove crucial for examining intrinsically unstructured proteins.
Another study carried out by Pepperkok et al (1999) sought to detect multiple green fluorescent proteins using fluorescence microscopy. The multiple gene fluorescence protein was being detected in live cells. The authors used fluorescence lifetime imaging microscopy to demonstrate how GFP variants had managed to yield discernible fluorescence lifetime. Those co-expressed GFP variants that demonstrated similar fluorescence intensities had to be separated using lifetime images. This enabled the researchers to open up extra spectroscopic dimensions thereby generating wavelengths that facilitated the selection of novel GFP variants.
Conclusion
Green Fluorescent Protein (GFP) is increasingly becoming popular in developmental and cellular biology, biotechnology, and microscopy because it has a phenomenal ability to produce noticeable fluorosphore. Shimomura et al (1962) first isolated GFP in 1962. Most bioluminescent coelenterates contain high levels of GFP. However, the hydrozoan jellyfish is the only known naturally occurring GFP molecule, while the Aequoria GFP genes are the only GFP genes to have been cloned thus far. GFP has a maximum absorbance peak at 295 nm. Photochemical observation of single GFP reveals an on/off switching and blinking display. The Ser-Tyl-Gly sequence acts as the originator of fluorosphore, which comes about owing to an auto-catalytic and thermosensitive reaction process. The basic structure of GFP entails an 11 stranded β-barrel that folds into the β-can structure. Multiple covalent interactions ensure that the barrel remains stable. Over the past few decades, GFP has found wide application in the fields of cell biology, microscopy, and biotechnology, among other fields. GFP is also increasingly being used in the discovery of new drugs owing to its ease of use and cost savings. As a result, it has now replaced other biological markets like β-galactosidase and luciferase. In the fields of microscopy, biotechnology and cell biology, GFP is being used as a reporter gene, as fusion tags, and as biosensors.
Reference List
Bastiaens, PIH & Pepperkok, R 2000, ‘Observing proteins in their natural habitat: the living cell’,Trends Biochem. Sci., Vol. 25, pp. 631-637.
Chalfie, M & Kain, SR 2005, Methods of Biochemical Analysis, Green Fluorescent Protein: Properties, Applications and Protocols, John Wiley & Sons, New York.
Chalfie M, Tu Y, Euskirchen G, Ward WW & Prasher DC 1994,’ Green fluorescent protein as a marker for gene expression’, Science, Vol. 263, pp. 802–805.
Chudakov DM, Matz MV, Lukyanov S & Lukyanov KA 2010,’ Fluorescent proteins and their applications in imaging living cells and tissues’, Physiol Rev., Vol. 90, pp. 1103–1163.
Creemers, TMH, Lock, AJ, Subramaniam, V, Jovin, TM & Volker, S 2000,’ Photophysics and optical switching in green fluorescent protein mutants. Proceedings of the National Academy of Sciences, Vol. 97, pp. 2974-2978.
Day, RN 1998,’ Visualization of Pit-1 transcription factor interactions in the living cell nucleus by fluorescence resonance energy transfer microscopy. Mol Endocrinol., Vol. 12 no. 9, pp. 1410-9.
Dickson RM, Cubitt AB, Tsien RY & Moerner WE 1997,’ On/off blinking and switching behaviour of single molecules of green fluorescent protein’, Nature, Vol. 24 no. 388, pp. 355-358.
Garcia-Parajo MF, Segers-Nolten GM, Veerman JA, Greve J & van Hulst NF 2000,’ Real-time light-driven dynamics of the fluorescence emission in single green fluorescent protein molecules’, Proc Natl Acad Sci., Vol. 97 no. 13, pp. 7237–7242.
Gerdes, HH & Kaether, C 1996,’ Green fluorescent protein: applications in cell biology’, FEBS Lett., Vol 389 no. 1, pp. 44-7.
Harpur, AG, Wouters, FS & Bastiaens, PI 2001,’ FRET between spectrally similar GFP molecules in single cells’, H. Nat. Biotechnol., Vol. 19, pp. 167-169.
Misteli, T & Spector, DL 1997,’ Applications of the green fluorescent protein in cell biology and biotechnology’, Nat Biotechnol., Vol. 15, no. 10, pp. 961-4.
Miyawaki, A, Griesbeck, O, Heim, R & Tsien, R 1999,’Calcium as a Cellular Regulator’, Proc. Nat. Acad. Science U.S.A., Vol. 96, pp. 2135-2140.
Ohashi, T, Galiacy, SD, Briscoe, G & Erichson, HP 2007,’An experimental study of GFP-based FRET, with application to intrinsically unstructured proteins’, Protein Science, Vol 16, pp. 1429–1438.
Pepperkok, R, Squire, A, Geley, S & Bastiaens, PIH 1999,’Simultaneous detection of multiple green fluorescent proteins in live cells by fluorescence lifetime imaging microscopy’, Current Biology, Vol. 9, No. 5, pp. 269-274.
Shaner NC, Patterson GH & Davidson MW 2007,’ Advances in fluorescent protein technology’, J Cell Sci., Vol. 120, pp. 4247–4260.
Snapp, EL 2009,’Fluorescent proteins: a cell biologist’s user guide’, Trends in Cell Biology, Vol. 19 No 11, pp. 649-655.
Shimomura, O, Johnson, FH & Saiga, Y 1962,’ Extraction, purification and properties of aequorin, a bioluminescent protein from the luminous hydromedusan’, Aequorea. J. Cell. Comp. Physiol., Vol. 59, pp. 223–239.
Tsien, RY 1998,’The Green Fluorescent Protein’, Annual Review of Biochemistry, Vol. 67, pp. 509-544.
Wouters, FS, Verveer, PJ & Bastiaens, PIH 2001,’ Imaging biochemistry inside cells’, Trends Cell Biol., Vol. 11, pp. 203-211.
Zimmer, M 2002,’Green Fluorescent Protein (GFP): Applications, Structure, and Related Photophysical Behavior’, Chem. Rev., Vol. 102, pp. 759-781.