For many proteins to be functional, the amino acid chain has to acquire a three-dimensional stable structure during the process of production within the cell. This is similar to twisting a wire to make a functioning tool (paper clip, a spring…). This progression is protein folding. Protein folding is, therefore, responsible for producing distinct functional units as enzymes and structural proteins used by the cell in many vital processes. Protein folding also precludes inapt interactions between proteins by hiding certain amino acid parts of the chain which if unhidden react indistinctively with other proteins (Miller, p. 1).
The endoplasmic reticulum is the cell stall where the synthesis of all proteins whether secretory; like hormones, antibodies, or enzymes, membrane proteins present on the cell surface as receptors. The endoplasmic reticulum is the cell compartment, which produces all other proteins that exist in the intracellular compartments like lysosomes. Thus, the endoplasmic reticulum provides a promoting location for building up and folding proteins. The endoplasmic reticulum as the name implies is a sequential chain of interrelated, intracytoplasmic, membrane-bordered pouches. The great number of ribosomes present along the surface of the rough endoplasmic reticulum produces proteins, which are then introduced into the lumen of the endoplasmic reticulum. Folding and assemblage of the new proteins take place in the endoplasmic reticulum aided by molecular chaperones and folding factors and sensors available. Chaperons unite with newly made proteins and disclose them to folding factors, which stabilize the protein structure through forming disulfide bonds. The folding sensors are responsible for finding out if proteins have the correct structure. If the correct structure is not obtained, protein recycling and repetition of folding take place, this is the calnexin cycle (Molinari, M, and colleagues, Pp.1397-1400).
Consumption of misfolded proteins can be crucial to the vitality of the individual; therefore, the endoplasmic reticulum possesses a quality control system. The function of this system is to make sure of the conformity and maturity of proteins produced by blocking the deliverance of incompletely folded or misfolded proteins, which are then destroyed in the cytoplasm. Both mechanisms of interrupting delivery and destruction (degradation) of misfolded proteins are not fully understood. Molinari and colleagues (Pp.1397-1400) suggested the presence of a mannose-binding lectin in the endoplasmic reticulum whose function is to regulate withholding of misfolded proteins. This lectin level affects the repetitive folding of misfolded proteins via the calnexin cycle; with high levels, earlier release of misfolded proteins from the calnexin cycle takes place with rapid degradation. In low-level lectin situations, prolongation of folding attempts in the calnexin cycle occurs, and degradation delays (Molinari, M and colleagues, Pp.1397-1400). Bukau and colleagues (Pp.443-451) suggested a monitoring chaperone (IRE1) which is a transmembrane kinase enzyme with a cavity (luminal) area to sense the misfolded protein. IRE1 is also a cytoplasmic kinase and a ribonuclease realm, figure 1 is a representation of how IRE1 recognizes unfolded proteins (adapted from Bukau and colleagues, p. 444). Hoffmann and colleagues (Pp. 22-29) suggested that degradation of misfolded proteins is through ATP-dependent proteolytic enzyme systems. Compared to bacteria, this is aided by a regulatory AAA chaperon which possesses adenosine triphosphatase (ATPase) action and an accompanying peptidase enzyme. In thermal stress, where misfolded proteins aggregate, this network does not work, and AAA chaperon does not escort peptidase but works with another system (DnaK system) (Hoffmann and others (Pp. 22-29).
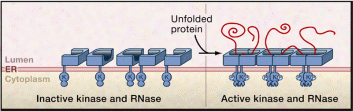
The word chaperone means to look after, guardian, or guide (Compact Oxford Dictionary), in this sense chaperone proteins are sequential protein chains present in the endoplasmic reticulum. These proteins guide the proper protein folding process via complicated binding and release processes (Pain, p. 365). Chaperones have some common features, they interrelate with unfolded or misfolded protein chains produced mainly from ribosomes (nascent), or extended enlarged chains transferred across organelles’ (subcellular) membranes. Chaperones; however, never form a part of the final protein molecule produced, they also assist in blending ATP (as an energy source) to the folding process. Chaperones are two types, nonspecific acting on various polypeptide chains, or specific aiming at certain protein chains. About shape, some chaperones are block-like, others are donut-shaped, others are tunnel-like, and others are made of paired subunits. There are two well-studied chaperone systems, Hsp70 (Heat shock protein70) and Hsp60 (Heat shock protein60); Hsp70 chaperones are many each has a precise function. Hsp70 chaperones work in association with co-chaperone proteins that adjust the chaperone activity. Examples of Hsp70 chaperones are DnaK (identified from E. coli bacteria), Ssa, and Ssb (identified from yeast). Hsp60 chaperones, occasionally called chaperonins are barrel-shaped that is formed of 14 to 16 subunits, each has an area of nonpolar amino acids that faces the inner wall of the barrel. Its function is to recognize the corresponding revealed nonpolar amino acids area of the misfolded protein. Examples of Hsp60 chaperones are GroEl (identified from E. coli), and TRiC/CCT (identified from eukaryotic cells). There are other systems of chaperone proteins, even nonbiological molecules as glycerol, chloride, urea, and NaCl can contribute as chaperons (Pain, Pp. 378-391).
The cellular regulatory process of protein folding includes molecular chaperones and protease enzymes that aid protein folding and scrutinize the quality of the ultimate protein product. Genetic or non-genetic factors may influence protein folding resulting in misfolded, destabilized, aggregated, or fibrillar protein formation. These are traits of the protein conformational disorders, where the altered protein molecular structures (conformations) result in cell toxicity or functional deficits. Examples of protein conformation disorders include neurodegenerative and metabolic disorders like Alzheimer’s disease, Parkinson’s disease, some types of encephalitis (spongiform encephalitis). Familial amyloid polyneuropathy, phenylketonurea (a metabolic disorder) are other examples. There are other neurodegenerative protein conformation disorders like Huntington’s disease, Creutzfeldt–Jakob disease. Cystic fibrosis of the lungs is also believed to be the result of protein conformation disorders. Various small chemical compounds such as osmolytes (osmotic pressure protectors as trimethylglycine), protein inhibitors, ligands (inorganic biological molecules). Some cofactors (nonprotein compounds connected to enzymes to form catalytic complexes) have a chemical chaperoning effect and can save folding and transfer defects; thus, minimize or partially surmount the pathological effects of protein misfolding (Leondrol and Gomes, Pp. 901-911).
Recent data lead to the conjecture that every polypeptide chain has an unknown yet existent beforehand concealed trait leading it to transform into a nonspecific toxin to cells in appropriate destabilizing conditions. This outlook of protein biology highlights the significance of the negative selection against molecules with a notable tendency to aggregate during the protein creation process. As well as during the biological process developing the complex molecular mechanisms striving for hampering misfolded proteins emergence and their toxic early aggregates. Chemical chaperones are on trial to reverse protein misfolding, small molecular mass molecules, as osmolytes (e.g. glycerol) are also on trial. However, chemical chaperones require high concentrations to produce an effect, while osmolytes are short of specificity. Experimental research data suggest repair of misfolded proteins represents a practicable therapeutic approach for protein conformation disorders. The use of chemical chaperones proved to have a little adverse effect in animal experimental studies. Chaperone treatment in humans still needs further studies to confirm efficacy and safety. Further understanding of the causes of protein aggregation, the genetic and environmental vulnerability of a certain individual will provide better prospects for effective therapeutic intervention (Stefani, Pp. 5-25).
Protein folding is still a major confounding problem of molecular biology. It is not only an academically attractive and challenging question, but also it is related to various applications and incapacitating diseases. Academic understanding of folding and assembly increased significantly during the past few years, particularly about cellular factors as chaperones, which are important for protein folding. Besides applying this knowledge witnessed notable progress; therefore, molecular, chemical, and pharmacological chaperones may change the mode of treatment and open a new frontier in clinical research of neurodegenerative diseases.
Works Cited
Bukau, B., Weiss man, J., and Horwich, A. “Molecular Chaperones and Protein Quality Control.” Cell vol 125 2006. p. 443-451.
Hoffmann, A., Kramer, G., Merz, F., Patzelt, T. et al. “Mechanisms of Chaperone and Proteases.” Bernd Bukau. 2005. Web.
Leonardo, P., and Gomes, C., M. “Protein Misfolding in Conformational Disorders: Rescue of Folding Defects and Chemical Chaperoning.” Mini-Reviews in Medicinal Chemistry vol 8 (9) 2008. p. 901-911.
Miller, N. “The misfolding diseases unfolded.” Beremans Limited. 2004. Web.
Molinari, M., Calanca, V., Galli, C., Lucca, P. and Paganetti, P. “Role of EDEM in the Release of Misfolded Glycoproteins from the Calnexin Cycle.” Science vol 299 2003. p. 1379-1400.
Pain, R. H. (Editor). Mechanisms of Protein Folding. Frontiers in Molecular Biology. New York: Oxford University Press, 2000.
Stefani, M. “Protein misfolding and aggregation: new examples in medicine and biology of the dark side of the protein world.” Biochimica et Biophysica Acta vol 1739 2004. p. 5-25.