Infrared radiation
Infrared radiation (IR) is a kind of radiation that lies on the electromagnetic spectrum between the visible and the microwave region, beyond the red light. Objects absorb and emit this kind of radiation with varying characteristics and intensities. Usually, IR is in various forms including thermal energy (Hsu, n.d). Typically, different bodies absorb and emit IR in different forms. Most bodies that emit the thermal radiation release this energy that can also be radiated at room temperature (Hsu, n.d).
It is worth noting that IR is absorbed due to molecular vibrations. It is important to note that molecular vibrations are caused by IR energy due to their rotational movements. Infrared radiation is categorized into various groups by the international commission of illumination. That is into Near-infrared where the level of absorption by water is low.
These characteristics suit the Near-infrared for us as image intensifier during night vision. The next classification is Short-wavelength infrared where water absorption levels increase significantly making them suitable for long distance data transmissions. The next classification is Mid-wavelength infrared that is suitable for use in guided missile technology followed by the g-wavelength infrared, and the far infrared laser suitable for medical applications. Other applications of IR include imaging such for use in digital cameras. IR envisages a complicated technology where IR is converted into electrical signals through a sophisticated mechanism. It is important to note that conduction forms one of the methods of IR transfer to the environment besides other mechanisms such as convection and radiation (Hsu, n.d).
The theory of infrared absorption details the behavior of molecules and constituent atoms at room temperature. Atoms at room temperature continuously vibrate but respond to incident radiation on the host molecules. That causes atoms to vibrate with greater vigor as incident radiation increases and when it is removed, the atoms settle back to their initial state releasing the energy they had absorbed in the process.
Infrared radiation spectrum
The infrared spectrum is divided into three categories. These include the near infrared which lies between the 0.76 to 1.5 microns, the middle infrared lying between 1.5 to 5.6 microns, and the far infrared lying between 5.6 to 1000 microns on the electromagnetic spectrum as illustrated in fig. 1 below.
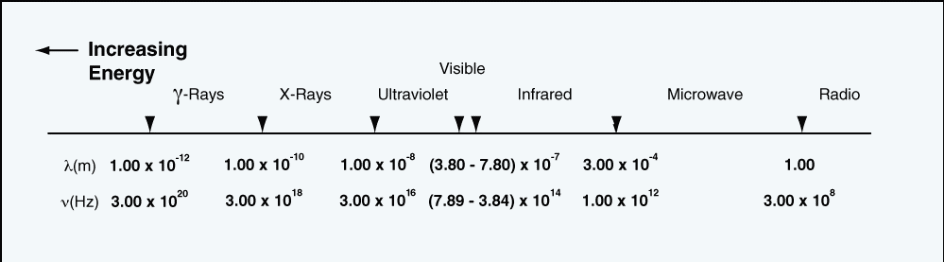
Mathematically, the energy due to infrared radiation is given in the relation,
E=hν = h(c/ λ) where E is the electromagnetic energy, h is Planck’s constant, c is the speed of light, and λ is the wavelength of the energy being considered. IR is therefore a function of the frequency of the incident radiation and the speed of light and the released energy (Hsu, n.d).
It is practically and theoretically proven that molecules absorb electromagnetic energy and release it into the surrounding when constituent atoms vibrate due to external excitations giving out a specific amount of energy by the molecules. However, the ratio of radiant energy from an object I0 is given by Transmittance, T, in the relation, A = log10 (1 / T) = –log 10T = –log10I/Io where A is the absorbance of incident radiation on an object. Mathematically, the transmittance spectra contrast between the intensities of radiation between of the three categories of infrared radiation that is Near IR, mid IR, and Far IR (Hsu, n.d).
The theory of infrared spectra details that molecules start to absorb incident radiation when the frequency of incident radiation is similar to that of IR (Hsu, n.d). Polyatomic molecules reflect a 3n degree of freedom allowing them to respond to non-linear vibrations in undergoing through translations and rotations when absorbing incident energy causing an IR activity in the molecules, the basis of absorbed radiation (Hsu, n.d). Typically, the vibratory nature of molecules in the IR region is characterized by stretches and bends. Typical examples of different modes of molecular vibrations are illustrated in the fig. 2 below.
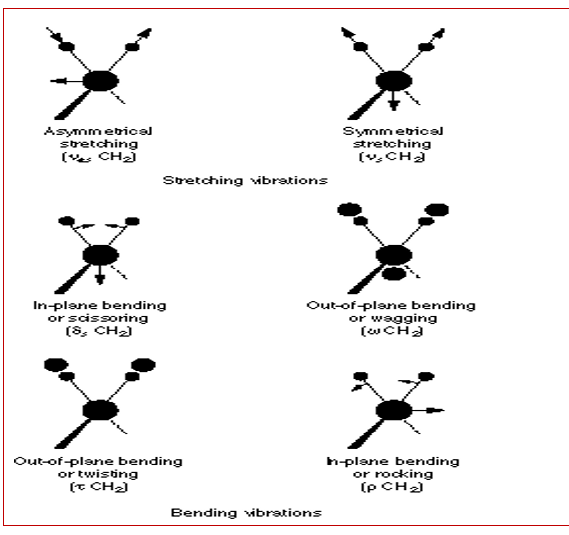
As mentioned above, the degree of freedom of a vibrating molecule is determined by the number of atoms that constitute the molecule. Mathematically, the degree of freedom is calculated based on the mathematical relation, 3n-6 for no-linear vibrations and 3n-5 for linear vibrations (Hsu, n.d).
Thus, when changes in transmittance occur with varying intensities, the IR spectra is obtained acting as the basis for the fundamental operation operational functionality of a number of instruments that are used in the medical industry (Hsu, n.d). These instruments include dispersive spectrometers and Fourier Transform Spectrometers among other designs in the industry today. A typical example of a spectrometer is illustrated in fig. 3 below.
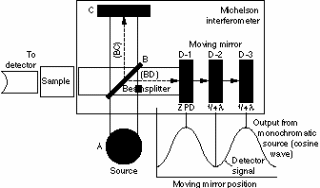
Operationally, a monochromatic source of radiation travels from source to the destination and any variations in intensity is detected by a sensor and recorded to reveal the structure of the intended target due to variations in intensity. That is particularly due to varying interference patterns hence underlying frequency characteristics (Hsu, n.d). It is important to note that different atoms and molecules exhibit different behavioral patterns on the IR spectrum. Therefore, different combinations of due to the molecular or atomic vibrations form the basis of IR spectrometry (Qi & Diakides, n.d).
Infrared radiation interaction with living systems
Living organisms or systems interact with IR and mounting evidence suggests a strong link between biological activities and the far infrared radiation. Typical evidence shows that IR inhibits the activity of cells in both humans and mice cells thus arresting any potential growth. That was particularly as a result of experiments that have been conducted to establish the relationship between the growth of cells and infrared radiation. A strong correlation between endogenous expressions and signal data as illustrated below yielded data that indicated a strong relationship between protein cells and their growth as illustrated below.
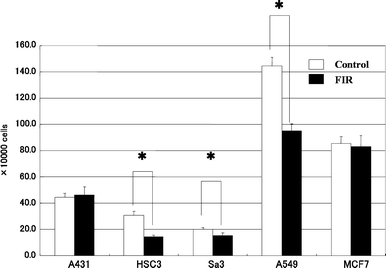
Infrared radiation in medicine
One of the typical applications of infrared spectrometry in the medical industry is in Medical Infrared Thermography (MIT). The infrared spectrometry is based on a physiological concept for detecting and controlling thermal abnormalities of the surface temperature of the human skin through thermal imaging of the skin surface. Areas of application include open-heart surgery, the reflex sympathetic dystrophy syndrome, and other medical conditions such as injuries particularly in athletic and related fields (Hsu, n.d).
MIT is a diagnostic tool that has been acknowledged by reputable medical associations such as the American Academy of Medical Infrared Imaging though the technology suffers from a lack of standards on reference thermograms used in diagnosis. However, research is underway to resolve the problem.
In principle, infrared spectrography relies on electromagnetic radiation frequencies to provide anatomical data on tissue physiology an approach characteristically non-invasive (Hildebrandt, Raschner & Ammer, 2010). Typically, the energy radiated from the human tissue acts as a basis for the classification of IR images which are variably related to the wavelength of the incident radiation on the skin. It has been demonstrated in theory and practice that at room temperature the human skin perfectly emits IR whose emissivity factor has been established to be 0.95 in the range of 2–20 µm. Some of the imaging modalities are illustrated in Fig. 4 below.
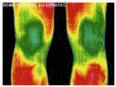
The specific wavelength of radiation used is IR, and the medical information gleaned from imaging the surface temperature provides detailed data of the anatomical physiology of the ankles.
Clinically, in Alpine skiing, is another typical example of the application of IR in the medical discipline where thermal images with temperature variations suggest physiologic abnormalities. In practice images are acclimatization and the resulting data from observations that are made using specific cameras are collected and analyzed. Modern technology uses specially designed software to analyze the collected data.
However, IR has its limitations. Though the technology is non-invasive, it has been identified to be a passive diagnostic method. On the other hand, a number of benefits have been identified salient with the IR technology. IR provides real time information about the condition of an injury, dynamic thermal imaging provides detailed information about skin stresses during performance and temperature changes during performances, and automated target identification in real time (U.S. Department of Health and Human Services Food and Drug Administration Center for Drug Evaluation and Research (CDER) 2003).
References
Hildebrandt , C., Raschner ,C., Ammer , k., 2010. An Overview of Recent Application of Medical Infrared Thermography in Sports Medicine in Austria. Web.
Hsu, C.-P. S. n.d. Infrared Spectroscopy. Web.
Qi, H., & Diakides, N. A. n.d Infrared Imaging in Medicine. Web.
U.S. Department of Health and Human Services Food and Drug Administration Center for Drug Evaluation and Research (CDER) 2003. Guidance for Industry Photosafety Testing. Pharmacology and Toxicology. Web.