A situation where visible light is absorbed is called the greenhouse effect.1 However, visible lights with longer wavelength are obstructed during the process. As a result, carbon dioxide (CO2) engulfs light rays of longer wavelengths.1 Thus, CO2 is an important factor that induces the greenhouse effect. In accordance with the Kyoto Protocol, it is a duty to reduce the emission of CO2. Thus, the aim of the greenhouse effect is to eliminate CO2 production or capture its release.
The channels for CO2 release include combustion and hydrogen production. However, it is possible to remove CO2 from the emission of combustion and hydrogen production.2 We must understand that the with the exception of controlled emission of CO2 and other gaseous substances, CO2 must be removed from gaseous streams.3 CO2 removal from gaseous streams includes ammonia synthesis and air separation.
The effect of global warming is increasing at an alarming rate. Fossil burning, plant emission and combustion engines are the primary source of greenhouse gas (GHG). As a result, GHG causes global warming. Energy demand from industrialized countries and the control of CO2 emission influence CO2 capture from gaseous streams. Documented report revealed that CO2 capture reduced emission from combustion and fossil plants by 90 percent.
Environmentalists established three routes for carbon capture. The methods include carbon synthesis and capture, cap market based control, and reduce carbon energy sources. However, on the basis of this article we will focus on the method of carbon capture and storage (CSS). In accordance with the Kyoto Protocol, CCS is a method of controlling the emission of CO2 from stationary plants. CSS techniques follow three stages.
The stages include CO2 detachment from gaseous streams, CO2 compression and storage. The process of CO2 capture is influenced by separation technique. As a result, CO2 emission is separated from gaseous streams, which include methane, and oxides of hydrogen, oxygen, and nitrogen. However, CO2 capture can be done in different ways, which include oxyfuel, post and pre combustion capture. The removal of CO2 emission from flue gas at high pressure is called post combustion capture.
Consequently, CO2 capture from syngas under high atmospheric pressure is called pre-combustion capture.4 However, oxyfuel combustion processes involve the reduction of CO2 emission from fuel combustion. The products of oxyfuel combustions are CO2 and water vapor. However, the processes of CO2 capture are cost efficient. As a result, more countries are experimenting cheap materials that can be used the same purpose. Thus, the discovery of efficient separation materials is a challenge for the CSS projects.
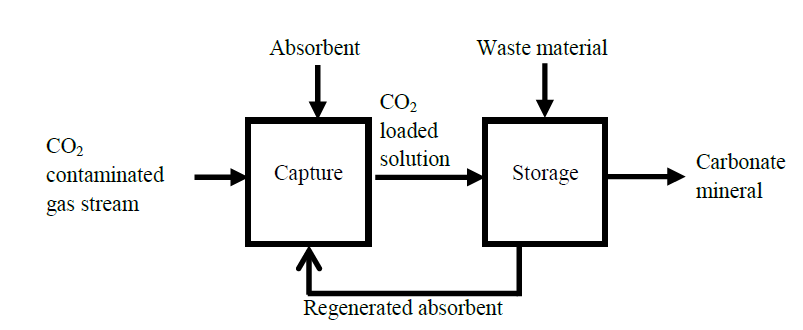
Porous materials
Porous materials are organic and inorganic polymers. However, organic materials have been widely accepted as porous materials. The features of porous materials include varying pore sizes, pore patterns, chemical compositions, and preparative approaches. Porous materials exist in two states, which include gaseous and solid state. However, porous metals are characterized by bulk density, ordered pores and independent.5,6,7,8
Consequently, metallic foams are characterized by low bulk density, and interloped pores. The cost of using the CSS technique influenced the discovery of porous materials for the remove of CO2 emission. As a result, the quality of the porous organic polymers is distinguished by its separation ability. Thus, the porous organic polymer used for CO2 capture must be CO2 specific, stable at extreme conditions with high affinity towards CO2.8 The quality of the porous material can be used in alkanolamine solutions organic polymers. The separation ability of the porous material influenced its use in alkanolamine solutions.
The aqueous alkanolamine technique involves the removal of CO2 using chemical absorption. As a result, gaseous streams are attached with aqueous alkanolamine solutions. The attached steam is purified at high temperature using aqueous alkanolamine solvent. The solvent removes CO2 from the gaseous streams. After extraction and compression, the porous material is regenerated for future use. Monoethanolamine is porous material used for CO2 capture. Another class of adsorbent materials is called porous organic polymers (POPs). These materials are produced using precise organic molecular building blocks (MBBs). The features of POPs include composition, function, control ability, and potential.
MEA can be used to achieve 90 percent efficiency. However, the material dissipates high amount of energy during regeneration. Thus, the absorption method remains the best techniques used in CSS projects. Solid adsorbent is another technology used for CO2 capture. The technology utilizes various adsorbent materials for CO2 capture. However, the process is influenced by the adsorbent material. Adsorbent materials are characterized by regeneration properties, adsorption capability, CO2 selective ability, and economic factors. The principles of adsorption can be separated by the adsorbent and the interface membrane.9
As a result, the adsorbent is attached to the surface of the adsorbed material. Adsorption process is a reversible technique. Consequently, adsorption processes exist in two phases. The physical process of adsorption is characterized by low temperature. However, chemisorption is characterized by with high temperature. Graphs are used to analyze the adsorption process. The study of graphs during adsorption is called isotherms.
Thus, isotherm can be studied in five different categories. Type I studies the adsorption process of micro porous pores. Type II isotherm studies the adsorption process of varying pore sizes. However, type III isotherm observes the adsorption process of monolayer formation. Type IV isotherm studies the adsorption process of two distinct layers. The layers include monolayer and the multilayer of the adsorbent. Finally, type V reveals the SVP during the adsorption process.
Adsorbent materials
Porous materials are classified according their pore sizes. As a result, the materials are classified as macrospores, mesopores, and micropores. Micropores materials include zeolites and activated carbons. As a result, different micro porous materials have combined in accordance with the specified requirement. The synthesized laboratory materials include MFOs, POPs, COF|s, CMPs, and HCPs. For the purpose of this study, we will analyze metal- organic frameworks (MOFs) and porous organic polymers (POPs).
Metal-Organic frameworks
MOFs are solid porous materials with a single ion, which are connected by organic ligands. Thus, MFOs structures are produced using MBBs requirements to ensure a porous network. The factors that affect the synthesis of MFOs structures include porous network, crystalline structure, ratio, nature of solvents, temperature, and concentration.9 The common building blocks used in MFO synthesis include carboxylate ligands, zinc and copper oxides. MFOs materials are characterized by structure, control ability, chemical composition and CO2 affinity.
Porous-Organic frameworks
POPs structures are synthesized from various organic monomers. POPs strategies include Sonogashira and ester production. POPs materials is characterized by thermal and chemical composition. Porous organic polymers can be classified into crystalline and amorphous structures. Crystalline structures are called covalent organic frameworks (CFOs). The synthesis of covalent organic framework is a reversible reaction.10 However, amorphous structures are characterized by irreversible reaction. The characteristics of amorphous structures include chemical stability, thermal capability and kinetic control. These features support post synthetic modifications.
Post-Synthetic modification
Post synthetic modification (PSM) describes the chemical change of the metal organic frameworks. The synthetic approach supports the functionalization and modification of MOFs structure.11,12,13,14 These structures are tailored in a heterogeneous fashion. As a result, PSM can be used to improve carbon activity, MOFS gas sorption and the catalytic activity. PSM has been used in different porous materials, which include activated carbon, zeolites and silica.
The adjustment of MOFs using post modification technique has been widely accepted. Thus, the modification of MOFs structures depends on post synthesis functionalization. Previous reports revealed that amine grafting influenced the CO2 affinity of absorbent materials. As a result, the stability of MOFs structure was achieved with previous experiments. Consequently, the functionality of amine grafting supports its international with CO2 capture using POPs and MOFs structures.155
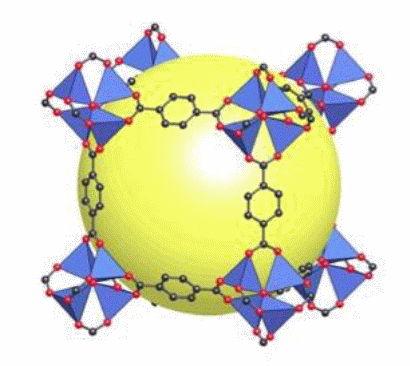
Finally, we analyzed the effects of different POPs structures using different amine grafting concept. Consequently, the mechanism of post synthetic modification was observed using In-situ design. We used fine characterization to analyze the structural qualities of the absorbent materials. Consequently, we prepared the porous organic polymers using numerous experimental methods. As a result, we qualified aldehyde-POP sing five techniques. The techniques include adsorption measurement (AM), Infrared Spectroscopy (IR), Elemental analysis, and themogravimetric analysis.16
We utilized POP, which offers stable results with post synthesis modifications. The first porous material was prepared with SH coupling. As a result, amine aldehyde was produced under normal conditions. The POPs structures include 1, 3, 5-triethynylbenzene (TEB), and Br2TMSBA. We will discuss the synthesis and characterization of aldehyde-POP using alkyne monomers. The materials used for the experiment were collected from Sigma-Aldrich. The list of solvents and chemicals include anhydrous N, N-dimethylformamide, which was stored with caH2, TEB (98%), Copper (I) iodide, and triethylamine. Other reagents include methanol, ethanol, ethylenediamine (EDA, 99%), diethylenetrimine, (DETA, 99%) and Tris-amine, 99%.
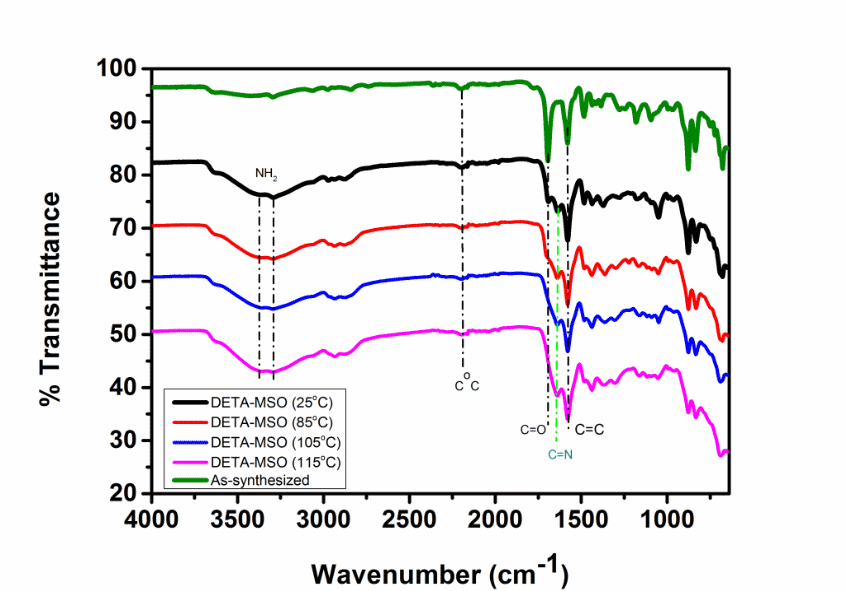
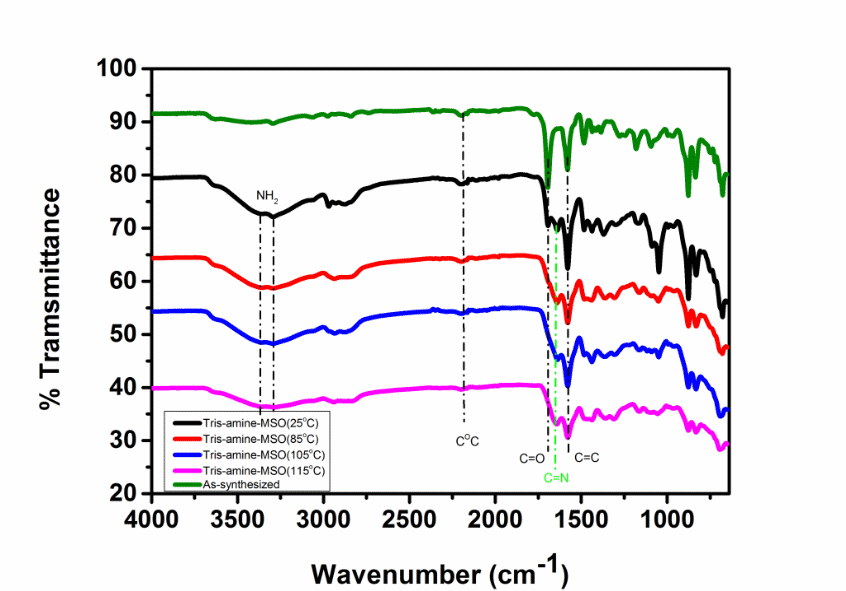
The synthesis was carried out on two monomers. The monomers include 2,5-dibromobenzaldehyde and 1,3,5-triethynylbenzene. However the monomers were catalyzed by bis(triphenylphosphine)palladium (II) dichloride and Cul. Consequently, the reactants were bubbled and stirred for twenty minutes with argon mixture. The mixture was heated fir twenty four hours at 80 degrees centigrade. After condensing the mixture, it was washed with different solvents. However, the solid residue was sterilized with ethanol for 24 hours. Upon release, the final product was heated at 60 degrees centigrade in an airtight vacuum. The calculated formula for the reaction is summarized below. C45H18O3. The percentages of each molecule of the element are 89%, 3.5%, and 3 present respectively.
The results were analyzed on the IR spectrum revealed a strong adsorption ascribed to the aldehyde group.1 Consequently, another IR spectrum showed a lone peak at 2160cm-1. The initial IR spectrum of 2, 5-dibromobenzaldehyde was absorbed at 1662.9cm-1. From the analysis, the first and second IR spectrum indicated a strong band at 1510cm-1, which corresponds to the benzene ring. Thus, the results indicated that a novel adsorbent material was synthesized. Finally, the synthesis and characterization of aldehyde-POP confirms the support for amine functionalization.1
The synthesis and characterization of amine grafted aldehyde-POP was conducted using different amine. The amine includes EDA, DETA and Tris-amine. We will compare the CO2 capture results from the three grafting amines.1 The initial experiment was conducted using a free aldehyde group, however, this experiment will be carried out with three different amine products. The synthesis of the amine grafting aldehyde was completed through the PSM grafting technique. Consequently, the conditions of the reactions were observed at using four distinct temperatures. As a result, temperatures were observed at 25, 85, 105, and 115 degree centigrade. The compound was administered with 10ml amine for one day. Consequently, the product was dried and filtered for 2 days.
The elemental analysis was computed for each amine group.
- EDA Aldehyde POP {[(C2) 3-C6H3] 2[C6H3-CHNC2H4NH2] 3} n
- C51H36N6: C=66.9 %( cal.83%), H=4.6 %( cal.4.9%), N=8.4 %( cal. 11%)
- DETA- Aldehyde POP {[(C2) 3-C6H3] 2[C6H3-CHN (CH2) 2NH (CH2) 2NH2] 3} n
- C57H45N9: C=66. 6 % (Cal. 80%), H=5 % (Cal. 5.3%), N=10 % (Cal. 14%)
- Tris-amine- Aldehyde POP {[(C2) 3-C6H3] 2[C6H3-CHN (CH2) 2N (CH2) 4(NH2) 2] 3} n
- C63H66N12: C=66 % (Cal. 70%), H=5. 5 % (Cal. 6%), N=10. 9% (Cal. 23%)
An IR spectrum was used to confirm the grafting of amine aldehyde-POP. However, amine attributed with EDA and DETA revealed single peaks. IR spectrum showed a single peak at 1690cm-1. The temperature range between 105 and 115 degrees revealed the peak disappearance. The report confirms the complete conversion of aldehyde-POP. The three grafted amine aldehyde had a similar vibration mode at 2160cm-1.
As a result, the amine structure was intact after the experiment. The characterization of three different amine were observed using the wavenumber, temperature, relative pressure, pole diameter, pressure bar and the amount of captured CO2. From the above result, we observed that the interaction between the amine group and the captured CO2 is significant. Thus, researchers must understand the mechanism for interaction. However, In-situ IR spectrum can be used to analyze these interactions during the adsorption experiment. From the experiment, it was revealed that under room temperature, CO2 accumulation and adsorption produces unstable groups.
There is a significant in spectra before and after the introduction of CO2. Consequently, the spectra shift to accommodate the increase in intensity. Finally, the result indicated the complete functionalization of aldehyde using three different amine grafting substitutions. Consequently, elemental analysis was used to analyze pore diameter and network. However, a reduction in the surface area of the compound was established from at the end of the experiment. In-situ IR spectra can be used to understand the interactions between the captured CO2 and the amine structures.
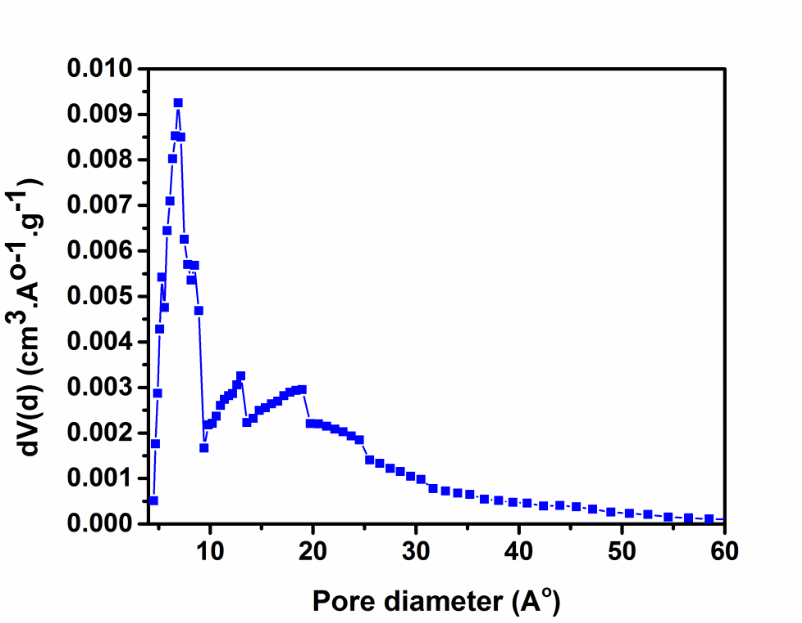
Conclusions
We synthesized porous organic frameworks through SH coupling using three different amine materials. Pore size, diameter, surface area, and volume were characterized in the reaction. Consequently, we conducted the modification of the aldehyde group using three amine structures. As a result, the functionalized structures revealed significant changes in Qst. In-situ can be used to analyze the interactions between CO2 and unstable species. Porous materials are organic and inorganic polymers.
However, organic materials have been widely accepted as porous materials. The features of porous materials include varying pore sizes, pore patterns, chemical compositions, and preparative approaches. Porous materials exist in two states, which include gaseous and solid state. However, porous metals are characterized by bulk density, ordered pores and independent. Finally, we analyzed the effects of different POPs structures using different amine grafting concept. Consequently, the mechanism of post synthetic modification was observed using In-situ design. We used fine characterization to analyze the structural qualities of the absorbent materials. Consequently, we prepared the porous organic polymers using numerous experimental methods.
References
- M. Eddaoudi, V. Guillerm, W. Alkordi, M. Mohideen, Y. Belmabkhout and A. J. Cairns, Chem. Commun., 2013. Web.
- M. Pera-Titus, Chemical Reviews, 2014, 114 (2), 1413-92.
- K. Sumida, D. L. Rogow, J. Mason, T. M. McDonald, E. D. Bloch, Z. Herm, T. Bae, J. Long, Chemical Reviews, 2012, 112 (2), 724-81.
- W. Lu, J. Sculley, D. Yuan, R. Krishna, Z. Wei and H. Zhou, Angew. Chem. Int. Ed., 2012, 51, 7480 –7484.
- A. Coˆte, A. Benin, N. Ockwig, M. O’Keeffe, A. Matzger, O. Yaghi, Science, 2005, 310, 1166-1345.
- J. Jiang, F. Su, A. Trewin, C. Wood, H. Niu, J. Jones, Y. Khimyak and A. Cooper, J. Am. Chem. Soc., 2008, 130, 7710–7720.
- A. Demessence, D. D’Alessandro, M. Foo and J. Long, J. Am. Chem. Soc., 2009, 131, 8784–8786.
- R. Woodward, L. Stevens, R. Dawson, M. Vijayaraghavan, T. Hasell, I. Silverwood, A. Ewing, T. Ratvijitvech, J. Exley, S. Chong, F. Blanc, D. Adams, S. Kazarian, C. Snape, T. Drage and A. Cooper, J. Am. Chem. Soc., 2014, 136, 9028−9035.
- N. Planas, A. Dzubak, R. Poloni, L. Lin, A. McManus, T. McDonald, J. Neaton, J. Long, B. Smit and L. Gagliardi, J. Am. Chem. Soc., 2013, 135, 7402−7405.
- T. McDonald, W. Lee, J. Mason, B. Wiers, C. Hong and J. Long, J. Am. Chem. Soc., 2012, 134, 7056−7065.
- F. Uribe-Romo, C. Doonan, H. Furukawa, K. Oisaki and O. Yaghi, J. Am. Chem. Soc., 2011, 133, 11478–11481.
- J. Jiang, F. Su, A. Trewin, C. Wood, N. Campbell, H. Niu, C. Dickinson, A. Ganin, M. Rosseinsky, Y. Khimyak and A. Cooper, Angew. Chem., 2007, 119, 8728 –8732.
- D. Beaudoin, T. Maris and J. Wuest, Nature Chemistry, 130, 334-678.
- F. Uribe-Romo, J. Hunt, H. Furukawa, C. Klock, M. O’Keeffe and O. Yaghi, J. Am. Chem. Soc., 2009, 131, 4570-4571.
- Z. Bacsik, N. Ahlsten, A. Ziadi, G. Zhao, A. Garcia-Bennett, B. Martín-Matute and N. Hedin, Langmuir, 2011, 27, 11118–11128.
- N. McKeown, S. Makhseedab and P. Budda, Chem. Commun., 2002, 125, 2780–2781.