Background of Grey Water Treatment
Pidou et al. (123) note that literature on the treatment of grey water has been there since 1970s. Membrane filtration accompanied with disinfection was among the primary technologies investigated for physical treatment. Biological treatment technologies like the aerated bioreactors rotating biological contactor and biological aerated filters were investigated in the 1980s and 1990s (Pidou et al. 123).
During the decade, the simple physical separation procedure fitted in residential places. At the turn of the millennia, MBRs as well as reed beds were introduced as alternatively sophisticated and cheaper technologies. Chemical treatment has also featured in literature, thereafter.
Figure 1: A diagrammatic representation of chemical technologies that applies filtration or flotation.
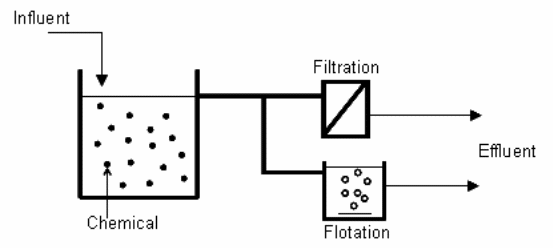
Source: Pidou et al. (30)
Figure 2: A diagrammatic scheme of a biological wastewater treatment system
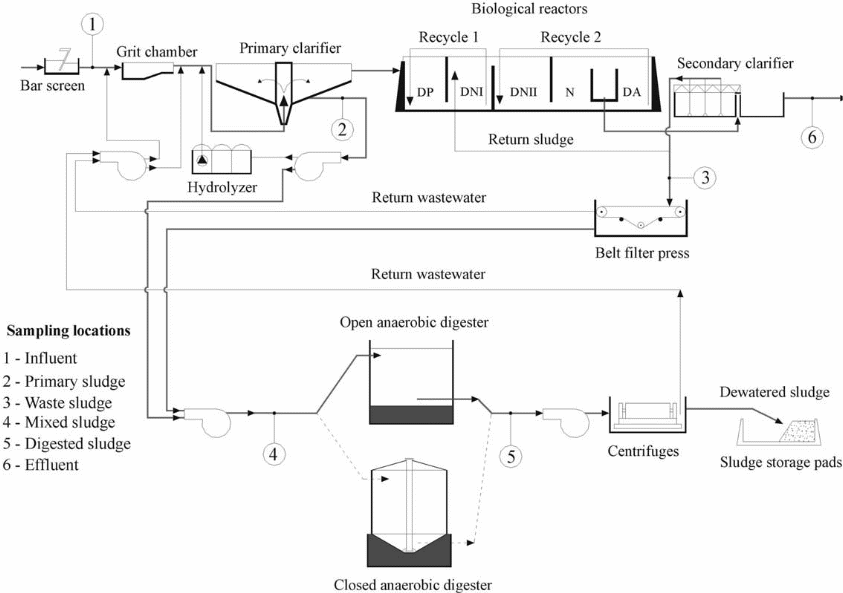
Source: Chipasa (137)
Description of Bio-Processes for Grey Water Treatment
Bio-processes in grey water treatment include fixed film reactors, rotating biological contactor, anaerobic filters, sequencing batch reactor, membrane bioreactors, and biological aerated filters (BAF) (Pidou et al. 18). Much of the literature reveals that in most settings bioprocesses hardly operated as standalone unless intended for pilot investigations.
Often, sedimentation, disinfection or screenings were installed as physical pre-treatment preceding the bioprocess systems (Pidou et al. 13). In other arrangements bioprocesses with membranes as in the case of activated carbon, MBRs, sand filters or extensive treatment technologies such as constructed wetland (Hastuti, Medawaty and Pamekas 368).
The Moving Bed Biofilm Reactor (MBBR) technology was a direct improvement of the Fixed Film Activated Sludge Process. At the onset of 1990s, Norway improvised the first MBBR. Thereafter, the corporate behind the invention began setting up small treatment units with design that integrated MBBR as well as the Norwegian State Pollution Control Authority recommendation.
In the recent past, there has been proliferations of large plants that apply the MBBR technology thus give the treatment method a big publicity in the wastewater industry. MBBR procedures are undertaken on bio-film systems within heavy carriers, which mix wastewater as well as suspend on the reactor with turbulence.
The system occurs between an activated sludge and an immovable bed bio-film system. MBBR boasts most of its strength from activated sludge coupled with bio-film carrier elements that are produced from polyethylene whose density falls below that of water. The elements are modelled to allow a lot of surface area for the bio-film.
MBBR treat incoming wastewater through microorganisms that are cultured on bio-carriers that suspend in the fluid within the MBBR reactor. The microorganism contained in the bio-film is heterotrophic in nature; thus, utilize organic carbon to accumulate new biomass (Abdul-Majeed et al. 1551).
Facultative bacteria are some of the highly preferred heterotrophs as well as serve to dissolve oxygen in the fluid composite. The viscosity of the biomasses rises with the increase of microorganism numbers within the bio-carriers.
The viscosity of the biomass impacts the strength of dissolved oxygen and substrate penetrating the bio-film. Those microorganisms on the frontiers of the bio-film have accelerated ability to mix oxygen and substrate through every layer in the array within the bio-film strata. MBBR have an aeration component that lowers present dissolved oxygen where the bio-film has aerobic, anoxic and anaerobic strata.
Nitrification in MBBRs has received rigorous research attention particularly with synthetic wastewater. Considering every bio-film reactors, nitrification rates are affected by the concentration of dissolved oxygen in the reactor, the concentration of cumulative ammonium nitrogen, organic load, the pH as well as alkalinity.
It was demonstrated that at the temperature of 15⁰ C and surplus cumulated ammonium nitrogen within an organic load of 1gBOD5/m2 biofilm surface area/d and a concentration of DO of close to 5 mg/L; this had a capacity to achieve a cumulative ammonium nitrogen rate of elimination of 1 g NH4–N/ (m2.d).
The quality of the water treated
Building complexes have resulted to use biological schemes in college hostels, stadiums and multi storey houses. This is tied to the bioprocesses efficiency on the Hydraulic retention times (HRTs) that falls between 0.8 hours and 2.8 days.
High strength grey water requires treatment systems that have higher HRTs such as mixed grey water and laundry wastewater whose BOD concentration ranges between 645 and 300-1200 mg.L-1. Compared to others biological systems perform fairly better at a mean time of 19 hours.
Such systems have organic loading rates for Chemical Oxygen Demand (COD) on the range of 0.10 and 7.49 kg.m-3.day-1; while for BOD varies from 0.08 to 2.38 kg.m-3.day-1 (Pidou et al. 14). In the case of the MBRs, organic loading rate values are lower at 0.88 kgCOD.m-3.day-1 compared to the standard wastewater treatment range of 1.2-3.2 kgCOD.m-3.day-1.
Stand alone systems applying the BAF, bio-films or rotating biological contactor (RBC) have their organic loading rate 1.32 kgBOD.m-3.day-1 thus their range falls within 0.3-1.4 kgBOD.m-3.day-1 (Pidou et al. 14).
Regardless of the range and type of the bioprocess, systems that integrate a biological component have efficacy in eliminating organic and other solids. Actually, there efficacy has been proved to be well above top range standards for reuse with residual results whose concentrations fall below 10 mg.L-1 (Pidou et al. 14).
An investigation into the turbidity concentrations achieved by biological systems, effluents residual have been positively below 8 NTU. Additionally, close to all schemes with bioprocesses achieved suspended solid residual under 15 mg.L-1. Bioprocesses performed better in the elimination of microorganisms by means for faecal coliforms and total coliforms at 5.2 log and 4.8 log, respectively (Pidou et al. 14).
At the remedial concentrations of 20 cfu.100mL– 1 through biological treatment, faecal and total coliforms remained below the stringent targets. An advantage of MBR as a bioprocess for treatment is its efficiency for the eliminating microbial without having a disinfection stage. To demonstrate this, Pidou et al. (15) achieved a 5 log and a residual concentration that is less than 30 cfu.100mL-1.
Other schemes employing MBR bioprocesses have given the robust elimination of the organic and solid residues at mean for BOD, turbidity and suspended solids at 3 mg.L-1, 3 NTU and 6 mg.L-1, respectively. However, the capability of bioprocesses treatment schemes are mildly impacted by changes in the strength and flow of grey water and potential shock loading (Pidou et al. 15).
Studies on the domestic product spiking on biomass resulting from an MBR showed that at EC50 the relative toxicity for perfume (at 2.5 mL.L-1), bleach (at 7 mL.L-1), washing powder (at 20 mL.L-1), caustic soda (at 23 mL.L-1) and vegetable oil (at 29 mL.L-1).
In other studies, the ability of MBR to revert to its initial performance capacity within a shorter period was indicative that MBR are minimally interrupted by feed, air or both; hence highly reliable. In the case when the feed was grounded for 25 days, no interruption was reported. However, there were different results when BAF was subjected to the same conditions.
With BAF, short interval stoppages had no performance implications, but longer led to rise in effluent concentrations and periods for recovery across factors. According to Pidou et al. (15), return to original-standards period as a result of feed stoppages lasting a third of a day were a sixth of a day for turbidity and TSS and close to two for coliforms.
Moreover, air stoppages lasting same period had a return to normalcy period were for BOD was 4 hours; for turbidity was 4 hours; for suspended solids was 24 hours; for faecal coliforms was 28 hours and for total coliforms was 24 hours.
When air and feed stoppages were both combined, the return to normalcy period lasted the longest were BOD was 40 hours; turbidity was 40 hours; suspended solids were 4 hours; faecal coliforms was 24 hours and total coliforms was 48 hours. None of the factors had regained the initial normalcy in less than two days when feeds were interrupted for 25 days.
Pidou et al. (16) observes there lacks adequate information on the cost for installation of bioprocess treatment systems. Previous studies on installation estimated a capital spending of £3,345 for systems that served 40 students boarding and comprised of a deep bed filter, a buffering tank, Granular Activated Charcoal (GAC) and an aerated bio-filter.
Spending on operation and maintenance on the consumables, energy and labor were annually estimated at £128. The return on investment was measured in terms of water savings with an annual estimation of £516 lasting over a period of 8-9 years (Pidou et al. 16).
It was projected that installing the system on a newly constructed house would cut the capital spending by £1,720 and readjust the period on return on investment by 4-5 years.
In a different bioprocess installation system that included a UV disinfection chamber, screening filter, a solids filter as well as a treatment tank on a bio-film generated by collection balls fitted to serve a household had a cost range between £2,514 and £3,325. Nevertheless, it was not immediately clear on the O & M cost as well as the return on investment details for the installation (Pidou et al. 16).
There are cheaper treatment installations done at a capital cost of about £197 that serve the housing of six persons that comprise of four plastic barrels. A treatment installation that had a sand filter, a UV disinfection chamber as well as a septic tank had a capital spending in £2,230 as well as an annual operating cost of £87.
The return on investment that recovered cost was annual water saving of £34 (Pidou et al. 16). About £30,000 will be capital budget for installing a bioreactor with a disinfector, sand filter and aerated for student resident wastewater treatment. The estimated annual spending for operations and maintenance was £611, while the return on investment through water savings was £166 (Pidou et al. 16).
Aqua-EMBR (Aquatech’s Enhanced Membrane Bioreactor) is a recent biological treatment technology applied at refineries and petrochemical settings. The treatment system has been effectively tried in the Middle East oil plants. The treatment system has further been enhanced via High Efficiency Reverse Osmosis capabilities to recover 90 percent high quality permeate.
The quality of the treatment meets the standards for recycling which is fed into the system for demineralising. Compared to the original MBR technologies, the Aqua-EMBR has some additional strength. Aqua-EMBR works without a membrane tank hence faster build up with reduced risks to the operators.
This technology has limited civil work requirements during installations, for instance can be mounted as skid on a level concrete slab. Operators and proponent face fewer risks in the event of delays posed by weather elements, environmental threats as well as other local eventualities during structural setting up (Al-Hamaiedeh and Bino 115).
Compared to submerged systems, Aqua-EMBR technologies are user friendly as well as convenient. The systems manager hardly senses the obnoxious smell or view or directly touches the bio-sludge (Xia et al. 7391). There are no exposed (from top) membrane tanks from where ambient air interacts with harmful aerosols.
In the event of need for operational checks and repairs, membrane modules can be replaced without direct contact with bio-sludge. This is not the case with submerged membrane modules mucked by sludge, hence creating chances for operators to get direct contact with when lifting out of the tank.
The system has flux that is higher by 50 percent which reduces the need for surface area vis-à-vis volumes permeate production by half. This lowers the capital spending and operational cost from the costing aggregation of membrane cost per unit volume filtrate. The Aqua-EMBR technologies provide footprint of lower than a fifth, which is the least known.
The technology promises cost savings by eliminating unnecessary man-hour attentions and chemical input costs. While contrasting with submerged systems, Aqua-EMBR technologies have reduced electricity needs by about 12.5 percent through the application of airlift pump effect.
The Aqua-EMBR technologies boast of the finest membrane pore type, which at the lower side are 30 nm and higher at 50 nm. The technology has a treatment efficacy of less than 0.2 NTU for turbidity and less than 0.5 mg/l for total suspended solid.
Advantages of Biological Grey water Treatment
Compared to other treatment technologies, bioprocesses are capable of eliminating organics. These technologies apply for only short retention times (Pidou et al. 18). While, other technology have a necessity for the disinfection stage to meet the standard for the elimination of microbial; MBR (a biological grey water treatment technology) does not need (Pidou et al. 18).
Pidou et al. (18) explains that the higher efficacy of technologies was observed when combinations of technologies were applied in a scheme. While, an aerated biological reactor alongside sand filter had a low strength treatment of grey water.
The scheme had GAC and disinfection retention concentration for BOD of 2 mg.L-1; a turbidity level of 1 NTU and total coliforms count of <1 cfu.100mL-1. Elsewhere, the combination of a disinfector, RBC as well as sendimentation filter has attended waster water from bathing with residual results of 0.6 NTU for turbidity; 5 mg.L-1 for suspended solids; 2 mg.L-1 for BOD and 1 cfu.100mL-1 for faecal coliforms (Pidou et al. 18).
At an individual level, only the MBR that had activated sludge and membrane had the capacity to proximate such results. A side-stream membrane bioreactor demonstrated residual results for treating grey water indicate a BOD of 1 mg.L-1; turbidity of 1 NTU; suspended solids of 4 mg.L-1 as well as total coliforms count of 1 cfu.100mL-1.
A submerged membrane bioreactor gave residual results with undetectable levels of suspended solids and coliforms; BOD of less than 5 mg.L-1 as well as turbidity levels below 1 NTU. Thus, it can be concluded that the MBR scheme is able to demonstrate residual results that meet the thresholds for reuse.
Notably, the level of treatment relies on the reuse application. According to Pidou et al. (20), biological treatment systems were found to have efficient hydraulic retention time of 19 hours over others.
MBBR allow choice of the filling of bio-film carriers in the reactor that allows the carrier to be suspended freely (Colic, Acha and Lechter 4150). The filling allowance extends up to 70 percent. The carriers are simply small plastic tubes with diameter and length of up to 5cm.
The production material is polyethylene with a density nearing g/cm3. The carrier is provided with several chambers that increase the total surface area of the bio-film. Thus, the features allow the treatment system to attend high strength industrial wastewater. MBBR surface area provides room for modification thus different shapes and size have been applied, while remaining effective.
It is imperative to assess the contribution of a water treatment to sustainable water management based on local settings as well as regional suitability. Though biological treatment technologies such as MBBR require substantively more energy investment to achieve a desirable carbon footprint, they are preferable to serve a wider area for quality grey water and economized energy vis-à-vis schemes developed from other technologies.
The advantages associated with MBBR include existing basins allow for increasing capacity, easily managed and operational; hardly do bio-film clog, no need for sludge return and enhanced nitrification for existing activated sludge facilities.
According to BP North America (35), benefits associated with MBBR include: no need for more acreage, has the capacity to eliminate phenol to an influent concentration of 200 mg/L, maintenance cost is manageable and has acceptable lifespan.
In addition, has robust tolerance ability to toxic shocks and insignificant sludge bulking issues as well as desirable eliminating capacity compared to other attached growth bioprocesses. Nevertheless, these bioprocesses have used a lot of energy.
Disadvantages of Biological Grey water Treatment
Extensive grey water treatment is the only technologies have more footprint than the rest. Biological treatment technologies provide limited footprint considering smaller spaces available in the urban environments.
There are schemes incorporating a rotating biological contactor, sedimentation tank as well a disinfection occupying a 15m3 volume that serves 70 occupants in a storey resident, hence servicing at a meagre 0.2 m2 per person connected.
Direct disadvantages associated with MBBR are elevated flows create potential loss of the element and the need for more stages to eliminate biological phosphorus. Moreover, there is a need for drive process to achieve more influent BOD loadings. MBBR achieve higher performance with extensive screening and removal or grit.
Analysis of Domestic Grey Water Mix
Grey water is a by-product the living habits of water consumers and hence the installations used in the due course result in variations in the ultimate characteristics of the water. The degree of variation depends on the intensity and diverse domestic uses resulting in grey and wastewater (Pidou, 9).
Analysis of the characteristics with reference to the type of household activity generating shows that kitchen and laundry sources produce more compared to others in terms of organics and physical pollutants. Arguably, kitchen wastewater accompanies food waste; while laundry wastewater has in its substantial contents of washing detergents (vis-à-vis its volume) (Pidou, 9).
Those households that do laundry outdoor the wastewater may contain in its sand and clay particles. Water from the bathroom (also referred to as the light grey water) contains mainly washing products and hair that are minimally concentrated as well as small proportions of organic materials.
The level of physical contaminants ranges between 33 – 249 NTU for composite grey water that is an indicator of high turbidity when compared to that of the sewer or potable natural water. The popular range particle size that cause turbidity occurs within 5 and 200μm.
Pidou (9) explain that particle size for various sources of water in bathroom fall in the range of 10-100μm; moreover molecules contained in grey water have molecular weight less than 3kDa. Grey water composite whose mean concentration level falls between 154 and 113 mg.L-1 for BOD as well as suspended particles, respectively, has concentration strengths that range between low to medium relative sewage (Pidou, 10).
Analysis of wastewater from bathroom falls within the low to medium strength of sewage; laundry falls at medium to high; while, kitchen sink at high strength sewage. The ratio of the chemical oxygen demand (COD) and the biological oxygen demand (BOD) estimates at 3.2. In most cases, domestic sewage and effluent discharge have their ratios at 2.2 and between 3 – 10, respectively (Pidou, 10).
The values reported for grey water imply that the biodegradability is relatively low. The COD:N:P ratio with values of 1:0.02:0.01 for grey water composite provided the necessary confirmation. Actually, the ratio reported for the same for sewage is at 1:0.09:0.02 (Pidou 12). It can be concluded the low rich nutrient of grey water contributes to the diminished biodegradability.
Analysis of waste water from bathrooms and laundry activities gives the ratio for COD:N:P at 1:0.02:0.06 and 1:0.02:0.15, respectively an indicator of the high concentration of phosphorus (Pidou, 10). The absence of N and P in the wastewater is because that comes from human waste. Pidou (11) explains that more than four-fifths and two-thirds domestic loads of N and P, respectively come from faeces and urine.
Pidou (11) explains that across the different wastewater types, the number of microbial ranged between 101 and 108 cfu/100mL. Moreover, added that the relative number of coliforms varies with the population distribution of residents found in the respective catchments (Pidou 12).
Interestingly, households that had no children had low total and faecal coliforms count at 80 and 6 cfu.100mL-1 compared to households with children at 3.2×105 and 1.5×103 cfu.100mL-1, respectively. Principally, investigations on grey water have established the presence of E. Coli, faecal coliforms and total coliforms as indicators of contamination.
There are likely chances of finding in grew water a variety of bacteria, protozoa, helminths as well as pathogenic viruses (Pidou 12). Hygiene and sanitation activities such as bathing of the young; after the toilet hand wash; doing contaminated apparel as well as rinsing edibles are the most likely contributors of microorganisms ending up in the grey water.
In a Swedish residential study, it was found that grey water had 101.4-104 cfu.100mL-1 for somatic coliphages as well as 102.3-104.8 cfu.100mL-1 for C. perfringes spores. The constituent for faecal enterococci was at 103-105.1 cfu.100mL-1; while the concentration of E. Coli and coliforms were 104.3-106.8 cfu.100mL-1 and 105.5-108.7 cfu.100mL-1, respectively (Pidou 12).
Heavy metals also accompany domestic grey water. These include zinc cadmium, nickel, copper, lead, tin, cobalt and chromium. Grey water has also been found to have high amounts of xenobiotic organic compounds (XOCs) (Pidou 12). It is highly advised not to discharge grey water after reuse due to toxic as well as environmental risks posed by the presence of xenobiotic organic contents in domestic ingredients.
There are higher chances of coming across XOCs in surfactants, preservatives and antioxidants, softeners, solvents, fragrances and flavours. The XOCs are not biodegradable, but also bio-accumulative.
There are bio-concentration factors more than 100 as well as a log Kow beyond 3. The toxicity with EC/LC50 is less than 1 mg.L-1. Pidou (12) cites that kitchen and laundry waters lead to higher toxicity levels in the domestic grey water.
Analysis of Oil Refinery Wastewater Mix
According to Ishak, Malakahmad and Isa (251) oil refinery activity require colossal amounts of water for desalting, catalytic and treatment procedures to generate desired outcomes, such as LPG, jet fuel, and petrochemical feedstock as well as distillation and thermal cracking.
It is estimated that the amount of wastewater generated is 0.4 to 1.6 times the quantity of unrefined oils. Nevertheless, release of untreated oil refinery wastewater into water masses poses environmental as well as human health impacts associated with harmful contaminants such as phenol, hydrocarbons, and dissolved minerals (Alade et al. 163).
Some hydrocarbons are carcinogenic. Persistent exposure to contaminants may lead to health complications like leukemia as well as tumor growths on organs. However, oil refinery wastewater has been treated using physical and chemical approaches, higher costs for chemicals and apparatus and massive sludge formation has made the biological approaches to be highly preferred.
Biological wastewater treatment methods are elementary, affordable as well as environmentally friendly. The application of membrane bioreactor achieved elimination efficacy within the range of 78 and 98 percent for COD, 96 and 99 percent for BOD, 74 and 99 percent for TSS and 99 to 100 percent for turbidity.
Table 1: Proportions of Water Use at the Domestic Level
Source: Pidou et al. (34)
Table 2: Distribution of Domestic Water Reuse
Source: Pidou et al. (36)
Table 3: The Characteristics of Domestic Grey Water
Adopted from Pidou (12)
Table 4: The Characteristics of Petroleum Refinery Wastewater
Source: Ishak, Malakahmad and Isa (252); Mahmoudkhani et al. (13)
Table 5: XOCs contributed into the domestic grey water
Modified from Pidou (12)
Table 6: Performance of MBBR Capacity on Conventional Sludge
Source: BP North America (28)
Table 7: Wastewater Parameters before and after MBBR Installation
Source: Colic et al. (17)
Table 8: Average Performance within a Month’s Period
Source: Colic et al. (18)
Assessment of Oil Refinery Wastewater Treatment Performance Across Biological Treatment Technologies
Source: Ishak, Malakahmad and Isa (254)
Table 9: The stringent Standards for Wastewater Reuse
Source: Pidou et al. (35)
Research theories and its application
MBR
According to Yang, Cicek and Ilg (201), research on membrane bioreactor technology has in the recent past concentrate on the UK, Japan and France, though the additional input has come from Germany, South Korea and China. There has been keen interest to integrate gas and extraction function within the MBRs solutions.
Scholarly works have delved on topical issues like fouling, optimizing operational performance and microbial characterization (Yang, Cicek and Ilg 201).
Other areas likely to benefit from MBR innovation include elimination of a nitrate treatment for drinking water, elimination of endocrine disrupting compounds from wastewater streams and water types, improving bio-fuels generation through the membrane aided fermentation and gas production and purification on MBRs (Yang, Cicek and Ilg 201).
MBBR
The recent development in biological wastewater treatment has several facets. The introduction of new technology of bio-film processes a derivative of activated sludge process under the theory of biological wastewater treatment.
Another face is the elementary process of wastewater treatment through processing system that result in biological transformation of organic matter such as the nitrification and de-nitrification.
There are advancement on the biological process of phosphorus elimination and impact mechanism. Other areas of research advancement are Activated sludge model series, bio-film model series, pollution sludge treatment process solutions.
Figure 3: Chronological account of Journal Artforle on MBR Technologies on Wastewater Treatment
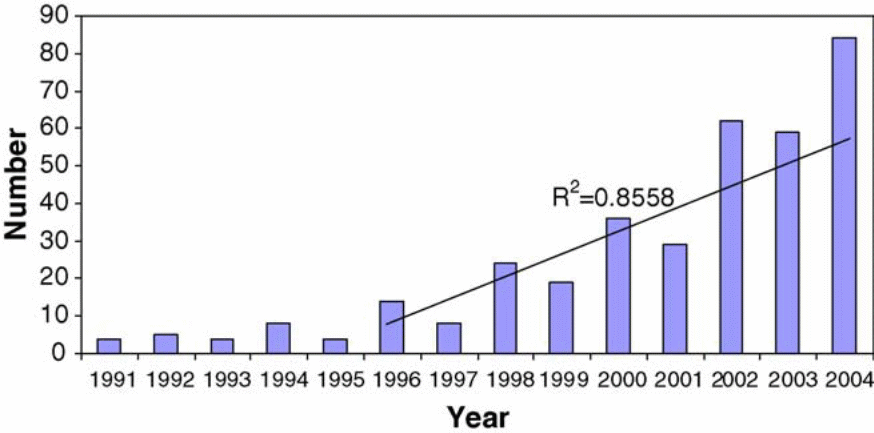
Source: Yang, Cicek and Ilg (202)
Figure 4: Chronological and Country Distribution of Journal Publications of MBR Technologies
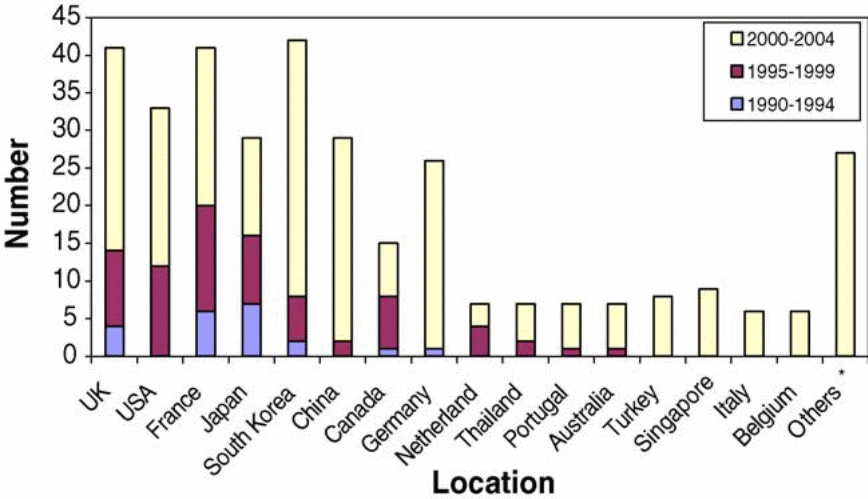
Source: Yang, Cicek and Ilg (202)
Table 10: Biological Wastewater Treatment Technologies Performance
Source: Pidou et al. (41); Al-Mughalles et al. (290)
Adaptation Of The Processes In Canada And North America
According to Yang, Cicek and Ilg (201), the application of MBR in North America occupies 11 percent of global installations. Popular brand of MBR in North America is Zenon, though elsewhere Kubota and Mitsubishi-Rayon are the common installations. It is focussed that the rise in stringent standards for wastewater treatment may popularize the MBR technologies, globally; thus driving innovation.
Figure 5: Distribution of Use of MBR Technologies
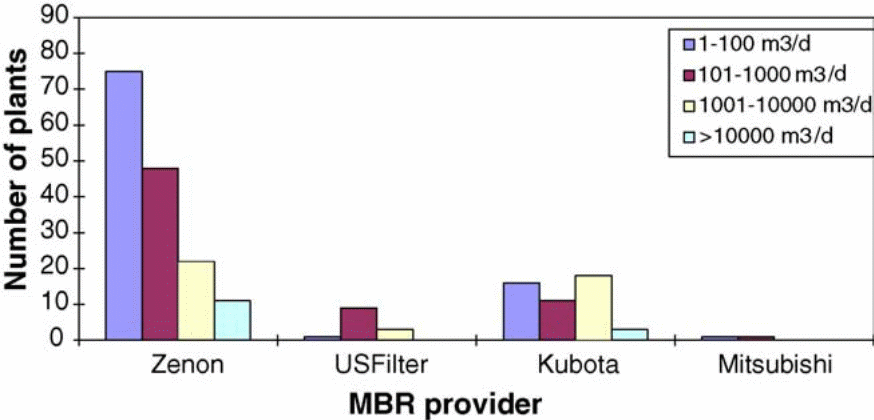
Source: Yang, Cicek and Ilg (207)
Conclusion
Literature show that the MBR technologies application has centred on wastewater treatment from domestic sources; while, MBBR technologies have been used in the oil refinery wastewater. Information on the advancement of MBR technologies is easily available vis-à-vis MBBR. This is probably, because the MBR technologies have present for longer than the MBBR ones.
The BP petroleum company in North America has comprehensively availed information on its application of MBBR technology, though no clear details on areas of technological improvement or future arrangement on the same.
Research interest in MBBR bioprocesses on wastewater treatment focus on enhancing the biological transformation of organic matter related to nitrification and de-nitrification. Research on MBR technologies relate to the elimination of nitrate, elimination of endocrine disrupting compounds, improving bio-fuels generation and gas production and purification.
Works Cited
Abdul-Majeed, Mohammed, Hisham Alwan, Mohammed Baki, Fauad Abtan and Hussein Sultan. “Wastewater Treatment in Baghdad City Using Moving Bed Biofilm Reactor (MBBR) Technology.” Eng. & Tech. Journal. 30.9 (2012): 1550-1560. Uotechnology. Web.
Alade, Abass, Ahmad Jameel, Suleyman Muyubi, Mohamed Karim and Zahangir Alam. “Removal Of Oil And Grease As Emerging Pollutants Of Concern (EPC) In Wastewater Stream.” IIUM Engineering Journal. 12.4 (2011):161-169. Iium. Web.
Al-Hamaiedeh, H and M Bino. “Effect of treated grey water reuse in irrigation on soil and plants.” Desalination. 256 (2010):115–119. elsevier. Web.
Al-Mughalles, Mohammed, Rakmi Rahman, Fatihah Suja, Mastura Mahmud and Sharifah Abdullah. “Greywater Treatment Using GAC Biofilm Reactor and Sand Filter System.” Australian Journal of Basic and Applied Sciences. 6.3 (2012):283-292. Ipcbee. Web.
BP North America 2008, Emerging Technologies and Approaches to Minimize Discharges into Lake Michigan. Web.
Chipasa, Kangala. “Accumulation and fate of selected heavy metals in a biological wastewater treatment system.” Waste Management. 23 (2003):135–143. Elsevier. Web.
Colic, Miroslav, Eric Acha and Ariel Lechter. “Advanced Pretreatment Enables MBBR Treatment of High Strength Candy Manufacturing Wastewater.” Water Environment Federation. (2009):4142-4152. Cleanwatertech. Web.
Colic, Miroslav, Wade Morse, Ariel Lechter, Jason Hicks, Steve Holley and Carl Mattia 2008, Enabling the Performance of the MBBR Installed to Treat Meat Processing Wastewater. Web.
Hastuti, Elis, Ida Medawaty and R Pamekas. “Application Of Domestic Wastewater Treatment Using Fixed Bed Biofilm And Membran Bioreactor For Water Reuse In Urban Housing Area.” Journal of Applied Sciences in Environmental Sanitation. 6.3 (2011): 367-376. Trisanita. Web.
Ishak, S, A Malakahmad and M Isa. “Refinery wastewater biological treatment: A short review.” Journal of Scientific & Industrial Research. 71 (2012):2521-256. niscair. Web.
Mahmoudkhani, Rouhallah, Akbar Azar, Alireza Dehghani and Hossein Ghoreishi. “Treatment of Contaminated Waters with Petroleum by Moving Bed Biofilm Reactor (MBBR).” 2012 International Conference on Life Science and Engineering IPCBEE. 45.3 (2012):12-16. Ipcbee. Web.
Pidou, Marc, Fayyaz Memon, Tom Stephenson, Bruce Jefferson and Paul Jeffrey. “Greywater recycling: treatment options and applications.” Engineering Sustainability. 160.ES3 (2007):119-131. dspace. Web.
Pidou, Marc 2006, “Hybrid membrane processes for water reuse”. PhD thesis, Cranfield Univ., 2006. Dspace lib. cranfield. Web.
Xia, Siqing, Liang Duan, Yonghui Song, Jixiang Li, Yvette Piceno, Gary Andersen, Lisa Alvarez-Cohen, Ivan Moreno-Andrade, Chun-Linhuang and Slawomir Hermanowicz. “Bacterial Community Structure in Geographically Distributed Biological Wastewater Treatment Reactors.” Environ. Sci. Technol. 44 (2010):7391–7396. Berkeley. Web.
Yang, Wenbo, Nazim Cicek and John Ilg. “State-of-the-art of membrane bioreactors: Worldwide research and commercial applications in North America.” Journal of Membrane Science. 270 (2006):201–211. elsevier. Web.