Research Proposal
The voyager interstellar mission was aimed at exploring the interface of the heliosphere with regard to the local interstellar medium. Voyager 1 crossed the termination shock of the solar wind in December 2004 and Voyager 2 crossed it in August 2007.
Data obtained from the V2 was used as a basis for the initial observations of the TS since V1 crossed the TS in a data gap. In the present time, the voyager spacecraft are taking measurements in the heliosheath, with V2 making the first plasma observations of the TS refion and HSH.
The Voyagers are the only spacecraft positioned to directly observe the boundaries of the heliosphere and have a chance to directly sample the LISM. These boundaries are the largest structures in the heliosphere and allow us to study physical processes such as magnetic reconnection, particle acceleration and transport, and the interaction of the solar wind plasma with the LISM neutrals in a system with scale size _100 AU.
These three topics, magnetic reconnection, particle acceleration and transport, and plasma-neutral interactions, are priority research focus areas identified by the SSSC roadmap.
The unique perspective of the Voyagers is crucial for these studies. The recent scientific discoveries bearing on these roadmap objectives are elaborated on in this proposal. They include the first crossings of the TS, the first observations of the HSH, verification of asymmetries in the heliospheric shape, and the lack of the expected ACR acceleration at the TS.
This paper looks at the Voyager Interstellar Mission, and the trips made by the voyages in the efforts to obtain information about the scale size and shape of the heliosphere. The first bit of this information was attained after the crossings of the TS. V1 was assigned to the northern hemisphere of the heliosphere.
It crossed the TS at 94 Astronomical Units while V2, in the southern hemisphere crossed it at 84 AU. The interpretation of this information was useful in evaluating the asymmetry in the TS crossing distances. This verified that the southern hemisphere of the heliosphere is pushed inward by the interstellar magnetic field (ISMF). It was necessary to have V2 cross the TS several times due to data gaps.
According to the shock structure observed by V2 there was a very dynamic shock; one crossing had the foot/ramp structure typically observed at quasi-perpendicular supercritical shocks. A second crossing was observed to catch the shock reforming, with two ramp-like structures.
An interesting observation that was made indicated that the thermal plasma was heated by a factor of 10-20 less than expected. Models and analogy to planetary magnetospheres suggested the HSH temperature would be 1-2 million degrees K and the electron temperature would be about 30 eV. The observed ion temperature was only about 100,000 K and the upper limit for the electron temperature is 3-4 eV.
Other observations made indicated that the plasma flow speed was surprisingly low at V1 and high at V2 and the pickup ions are strongly heated. None of the foregoing observations were anticipated, which implied that these and future revelations of the unexpected nature of the HSH, HP, and LISM would drive theoretical modelling toward a new paradigm. The increase in solar activity will assist in the study of how interplanetary coronal mass ejections (ICMEs) and merged interaction regions (MIRs) propagate through and affect the HSH.
The study will involve observation of the recovery of cosmic rays beyond 100 AU at solar minimum, determination of the effects of a negative magnetic polarity solar cycle, and monitoring of the unfolding of the low-energy anomalous cosmic ray (ACR) spectra as V1 and V2 move through the HSH.
Introduction
The Voyager spacecraft were launched in 1977. The launch was on a trajectory toward the giant planets, which also happened to be toward the upstream direction of the heliosphere. The Voyager Interstellar Mission had successful planetary encounters and, therefore, it progressed outward with the view to making the first observations of the LISM. Both Voyagers have now crossed the TS, so the goal of reaching the LISM seems achievable.
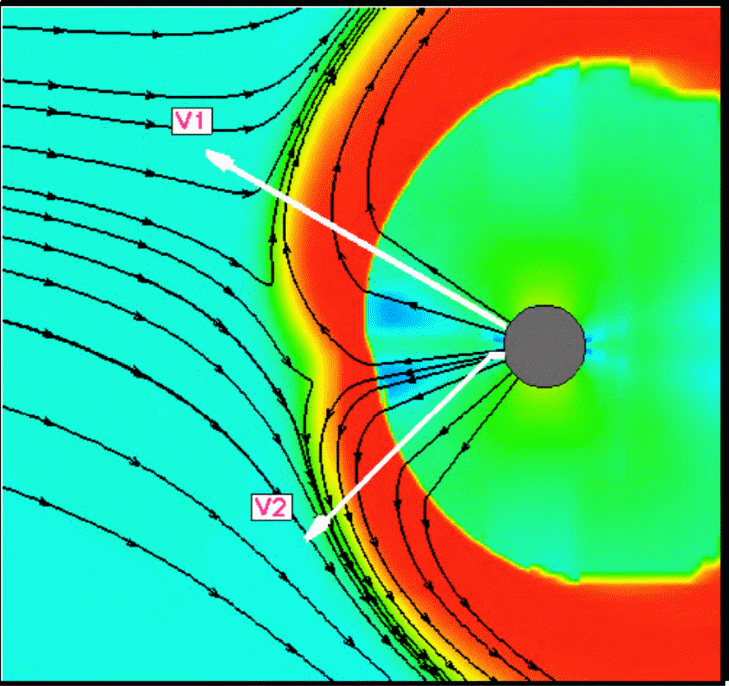
Figure: model of the heliosphere with the plasma flow lines superposed. The color scale shows the magnetic field magnitude. The white lines show the Voyager trajectories (McComas 243).
The solar wind moves outwards from the sun before it becomes supersonic near the Sun and moves at a nearly constant speed radially outward to 80-100 AU. At this point, the solar wind encounters the TS and becomes subsonic. The shocked SW in the inner HSH region extends outward to the HP.
The LISM plasma flow diverts around the heliosphere at the HP boundary, and the shocked solar wind rotates to flow toward the heliospheric tail. This subsonic flow of solar plasma is affected by the LISM, which we think is essentially uniform on the relevant _100 AU spatial scales and decade-long time scales (McComas 241). The V1 crossing of the TS set the scale for the whole heliospheric system. The missing piece before this crossing was the pressure in the LISM.
Knowing the TS boundary distance fixes this parameter. Models predict that the HP is 30-50% more distant than the TS. The V2 crossing of the TS tells us about the asymmetries in the heliosphere; the boundaries in the south are closer to the Sun than those in the north (McComas 244). On their way to the LISM, the Voyager spacecraft explore the HSH. This region of subsonic flow is very active, as indicated by the large fluctuations in the plasma and magnetic field over times scales of hours to days.
These variations are due to structures entrained in the solar wind and from those generated by the motions of the TS and possibly also the HP. Large fluctuations in MeV particle fluxes are observed but not yet understood (McComas 244).
The Voyagers are the outermost spacecraft in NASA’s heliospheric network, the ensemble of spacecraft collecting data in the heliosphere to provide a global picture of heliospheric processes. Voyager plays a critical role, as it provides direct observation of ACRs near their source region and of GCRs before they are modulated in the solar wind.
Comparison of 1 AU and Ulysses data with Voyager data has provided tests of models of solar wind evolution. Understanding how changes in the solar wind propagate through the HSH is beneficial since it help to understand and differentiate the effects of shock motion and solar wind changes on the HSH (McComas 245).
The Voyagers observe the integrated effects of solar wind evolution and interaction with the LISM from the inner to outer heliosphere. The various sections addresses by the voyager spacecraft are discussed in the sections that follow.
Termination Shock Characteristics
Termination Shock Particles (TSPs)
TSPs are approximately 30 keV to a few MeV/nuc ions which are accelerated locally at the TS and/or in the HSH and then escape into the upstream solar wind. V1 began measuring high intensities of >40 keV ions and >350 keV electrons in the solar wind upstream of the TS in July 2002 at a distance of 85.2 AU and a heliographic latitude of 33.8◦N. V2 at a heliolatitude of 27.5◦S entered the TS foreshock region in Dec. 2004 at 75.2 AU. This difference in location is qualitatively consistent with models which predict both a thicker foreshock region in the direction of V2 and that the TS is closer to the sun in the south than in the north (Szabo 61).
Energetic particles enable remote sensing of conditions at the particle source and in the plasma between the source and the spacecraft. Thus the upstream TSPs can tell us about the global structure of the heliosphere (Szabo 61). These important observations include:
- the locations of the particles
- their angular distributions, and
- The spatial and/or temporal evolution of their energy spectra, especially at lower energies.
TS Structure: Initial V2 Results
V2 first crossed the TS in a data gap on day 242 of 2007. The TS then moved outward past V2 on day 243. V2 remained in the solar wind for about 3.5 hours, and then the shock moved inward past V2. Three hours later another crossing occurred just before a data gap; since the end of day 244, V2 has remained in the HSH. The first solar wind signature of the TS was probably the speed decrease on day 160, 82 days (0.8 AU) before the TS crossing. A second speed decrease occurred on day 192 and a third on day 230 (Szabo 62).
The solar wind speed decreased from 400 to 300 km/s before the TS crossing, so almost half of the solar wind flow energy is removed and presumably transferred to energetic particles and/or pickup ions upstream of the TS (Szabo 62). We need to quantify and understand this process.
One hypothesis is that the flow energy heats the TSP ions. The first 2 of the step-like energy decreases before the TS were associated with MIRs. Another hypothesis proposed for the pre-shock solar wind slowdown is that hot electrons in the TS foreshock greatly increase the ionization rate of neutral interstellar hydrogen and that acceleration of these pickup ions slows the solar wind. V2 and Ulysses plasma measurements established that the solar wind slows down gradually because of mass loading from pickup ions created by charge exchange and photo- ionization of neutral interstellar hydrogen (Szabo 62).
Prospects for More TS Crossings
The variation in the TS distance is caused mainly by changes in the solar wind dynamic pressure. The solar wind dynamic pressure varies over a solar cycle. The solar wind dynamic pressure increases by a factor of _2 over a 1-2 year period just after solar maximum, then decreases slowly over the next 9-10 years before increasing again. Based on recent Wind data from 1 AU, the solar wind pressure decreases over the past year; are reaching the TS and it should, therefore, move inward (Kallenbach, Czechowski and Hilchenbach 203).
Heliosheath Physics and Structure
The inner HSH is the region between the TS and the HP. The in situ exploration of the HSH began when V1 crossed the TS on 16 December, 2004 and will continue until V1 and V2 cross the HP and enter the LISM. The flows and magnetic field in the HSH are strongly affected by:
- the solar wind, pickup ions and the interplanetary magnetic field in the supersonic solar wind;
- the neutral H atoms from the LISM;
- the interstellar magnetic field; and
- The ionized component of the interstellar medium.
The structure of the HSH is expected to vary with the solar cycle, as the consequence of varying solar wind input conditions to the HSH (McComas 249).
Heliosheath Size and Shape
Asymmetries of the TS and HSH could be introduced or modified by 3-D unsteady phenomena. For example, if a global MIR (GMIR) or another large solar wind disturbance were not spherically symmetric, it could introduce a substantial asymmetry in the TS.
The asymmetry observed by the Voyager spacecraft helps derive important information about the solar wind and LISM properties. Only continued observations by V1 and V2 can determine the relative importance of the ISMF effects, charge exchange, and transient phenomena that control the size and shape of the HSH (Kallenbach, Czechowski and Hilchenbach 203).
Heliosheath Composition
The LISM surrounding the heliosphere is weakly ionized. The interaction between the LISM and the heliosphere is crucially affected by charge exchange between neutral and charged. Charge exchange influences the flows within both the supersonic solar wind (solar wind) and the outer HSH.
The solar wind is decelerated by mass loading of PUI as it moves toward the TS. The PUIs are hot, dominating the internal pressure of the solar wind beyond 20 AU; therefore PUIs strongly influence the solar wind dynamics. Charge exchange decreases the heliocentric distances of the TS, HP, and BS. In the outer HSH, the LISM protons decelerate when they approach the HP.
This deceleration results in a difference between the velocity of protons and neutral H-atoms, leading to enhanced charge exchange which slows down the H and produces a layer of increased H-atom density (a “hydrogen wall”) in the outer HSH.
Large-scale Flow, Internal Energy, and Magnetic Field in the Heliosheath
The plasma flow velocity as a function of position is a fundamental characteristic of the HSH. In lieu of plasma data from V1, the LECP team used angular data from low-energy ions to estimate the projection of the plasma flow velocity onto the RT-plane. Since the magnetic field is frozen-in to the plasma, a rotation of V as V1 (or V2) moves toward the HP implies a corresponding rotation of B. Both the magnetic field and velocity should be parallel to the surface of the HP on average, at the HP (McComas 249).
Solar Cycle Variations
Models of the HSH thickness suggest that the Voyager spacecraft will spend the better part of a solar cycle in the HSH and will be able to study the time evolution of the HSH. In situ observations at 1 AU during 2007 show the presence of corotating streams. The solar wind takes roughly one year to propagate from the Sun to the Voyager spacecraft. As the solar cycle progresses, CMEs and the associated transient flows in the solar wind will become more frequent; interaction regions will merge to form MIRs, which are often preceded by shocks.
When these shocks and MIRs arrive at the TS, they push it outward as they propagate through the TS into the HSH. These shocks might produce an increase in the HSH speed, density, temperature, and magnetic field at V1 and V2 (Moraal, Caballero-Lopez and Mc- Cracken 219).
Turbulence, Fluctuations and Small-Scale Structure in the Heliosheath
Turbulence and other fluctuations in the HSH might be driven by a number of processes including magnetic reconnection, anisotropic particle distributions, plasma processes associated with the heliospheric current sheet and the enhancement of solar wind turbulence as it crosses the TS (Moraal, Caballero-Lopez and Mc- Cracken 219).
Anomalous Cosmic Rays and Acceleration
Anomalous Cosmic Rays
Before the V1 TS encounter in late 2004, it was assumed that ACRs were accelerated at the TS by diffusive shock acceleration (DSA) to energies 1-300 MeV/nuc. After 2004, both the V1 He spectrum in the HSH and the V2 spectrum upstream of the TS continued to evolve toward the expected source shape.
After the V1 TS crossing, several models emerged to explain the deficit of ACRs at mid energies. According to scientists, the stochastic acceleration in the turbulent HSH continues to accelerate ACRs and the high-energy source region is, therefore, beyond the TS (Moraal, Caballero-Lopez and Mc- Cracken 219).
The evolution of the ACR spectra at both V1 and V2 as a means to discriminate among the different models for the ACR source will continue to be observed over the years. The ACR observations will allow a more definitive determination of the composition of ACRs and of their propagation mean free path in the HS (Moraal, Caballero-Lopez and Mc- Cracken 219).
Acceleration
An essential aspect of ACR acceleration, particularly by DSA at the TS, is injection. As interstellar neutral atoms are continuously ionized and picked up by the solar wind, their initial velocity-space ring distributions are isotropized to shell distributions before being cooled adiabatically to roughly uniform spherical distributions.
These atoms are also accelerated as they convect outward toward the TS. The relatively high-energy extents of the pickup ion velocity distributions enable them to be injected more easily into the DSA process at the TS. Stochastic processes operate over long periods of time as pickup ions convect with the solar wind and typically produce exponential tails with e-folding speeds that are sensitive to scattering coefficients and particle species (Parker 29).
Galactic Cosmic Ray Modulation
A study of the unexplored region of solar modulation beyond the TS not under the influence of the outward flowing solar wind with the resultant adiabatic energy loss is aimed at the determination of the interstellar cosmic ray spectra for all species, the primaries such as H, He, C, O, Fe, secondaries such as B and N, and electrons.
The spectra, charge and isotopic composition of these different species, all of which the CRS experiment can accurately measure, contain a wealth of information about conditions locally and on a larger scale in the galaxy itself. The plasma density, magnetic field, and the structure and turbulence on large and small scales that determine the actual propagation (diffusion) of these particles throughout the galaxy will be accessible to measure (McComas 249).
Bearing this in mind, the Voyager can be said to be a true interstellar probe, with the ability to study astrophysical features of the galaxy that have been previously inaccessible to direct measurement.
The Heliopause And Beyond
In Situ Observations
Now that both Voyagers have crossed the TS and are in the HSH, the next boundary to be sought is the HP. Unlike the case in the solar wind upstream of the TS, the HSH flow is subsonic and the plasma flow speed and magnetic field in the HSH will be affected by the location and nature of the HP. Measurements of the plasma, the magnetic field, the energetic particles, the cosmic rays and the radio emissions will help us understand the nature of the interaction of the heliosphere with the LISM.
Once the HP is crossed, the Voyagers will make the first in situ observations of the interstellar medium, adding immeasurably to our understanding of the interstellar medium and the nature of our galaxy. The neutral component of the LISM plays an important role in this interaction, even though it cannot be directly measured (Parker 31).
The size and shape of the heliosphere depend on the properties of both the solar wind and the LISM. Among the several physical quantities that describe the interaction of the solar system with the LISM, the least known are the direction and intensity of the ISMF. Models suggest that the strength of ISMF is a few microgauss.
The interstellar magnetic field is frozen into interstellar plasma that is deflected around the HP, causing the field to drape over the HP. If the plane of the interstellar magnetic field were not in the meridional plane of the sun and the angle between the ISMF and interstellar velocity were non-zero, magnetic forces would break the symmetry of the heliosphere (Parker 32).
These magnetic forces would cause a distortion of the HP and the TS. For magnetic field intensities of a few microgauss, the ambient interstellar magnetic stresses are comparable to the gas pressure. The major HSH signatures the approaching HP should be a deflection of the plasma flow and an increase in the plasma density.
In situ observations of the magnetic field from both V1 and V2 should provide additional information concerning the flow patterns and the location of the HP, although the large fluctuations in the magnetic field may mask some of these effects. We expect that the magnetic field magnitude will increase significantly when the Voyagers cross the HP, since the ISMF should be significantly larger than the extended interplanetary magnetic field at the HP (Parker 33).
In analogy with planetary magnetopauses, the HP is likely to be a complex surface that varies locally in thickness and orientation and is the site of processes such as patchy reconnection and a variety of plasma instabilities. Intermittent reconnection along a slightly corrugated HP surface might appear in the low-energy ion (and possibly electron) intensity-time profiles as gradual intensity increases with superposed, anisotropic intensity spikes as the spacecraft approaches the HP, similar to the structures encountered in front of the TS (Parker 33).
Radio Emissions
For over twenty-five years the plasma wave instruments on the two Voyager spacecraft have detected sporadic radio emissions from the outer heliosphere in the frequency range from about 2 to 3 kHz. The source of these emissions is not well understood, although the observed radio emission frequencies (2 to 3 kHz) could only be produced at or near the HP.
Shocks have been hypothesized to produce radio emission via energetic electron acceleration at the shock. Models have indicated that the source line is perpendicular to the projection of the ISMF onto the HP. This model is based on the fact that electron acceleration at an interplanetary shock is highly sensitive to the magnetic field direction and is strongest when the magnetic field is nearly perpendicular to the shock normal (Szabo 68).
Conclusion
The Voyager spacecraft continue their epic journey of discovery, travelling through a vast unknown region of our heliosphere on their way to the interstellar medium. V2 has just crossed the TS and provided many scientific surprises. Both Voyagers are now traversing the HSH, with the first crossings of the HP and the first in situ observations of the interstellar medium still to come.
These encounters could answer many basic, long-standing questions about the plasma and magnetic properties of the LISM, the nature of the TS and its role in the acceleration of the ACRs, the role of the HSH in GCR modulation, the spectra of low-energy interstellar GCRs, and the source and location of the heliospheric radio emissions (McComas 250).
Exploratory missions such as Voyager provide key tests of physical theories and also provide observational surprises which often lead to major advances in physical understanding. The energetic particle beams observed upstream of the TS are certainly an example of such a surprise which has revised current thinking on the morphology of the TS surface.
The continued ACR modulation in the HSH, the pre-shock slowdown and lack of heating of the thermal plasma at the TS, the low shock strength of the TS, and increases in the 6-14 MeV galactic electron intensities in the HSH are other examples forcing revisions to long-term hypotheses on particle acceleration (McComas 250).
The longevity of the Voyagers makes them ideal platforms for studying long-term solar wind and now HSH variations. Their distance makes them ideal for studying the evolution of the solar wind, shocks, and cosmic rays. The interpretation of Voyager data is greatly enhanced by the ability to compare with data from Earth-orbiting spacecraft (Wind, ACE, SAMPEX, STEREO), Ulysses, and in the near-future IBEX. These data make deconvolution of solar cycle, distance, and latitude effects possible (McComas 250).
Works Cited
Kallenbach, R., et al. “Physics of the Heliospheric Boundaries.” ISSI Scientific Report No. 5 (2006): 203. Print.
McComas, D.J. “Physics of the Inner Heliosheath: Voyager Observations, Theory, and Future Prospects.” Annual IGPP International Astrophysics Conference. (2006): 858, 241-250. Print.
Moraal, H., et al. “Cosmic ray energy changes at the Heliosheath.” (2006): 219.
Parker, E. “Interplanetary Dynamical Processes.” Interscience (1962). Print.
Szabo, A. Multiscale Processes in the Earth’s Magnetospher. From Interball to Cluster, edited by J.-A. Sauvaud and Z. Nemecek 57-71. New York: Springer, 2004. Print.