Introduction
A chemical laser is a powerful device that directly converts chemical energy into coherent radiation. Their fields of application are mainly military tasks, for example, as anti-missile weapons, which will even work onboard large aircraft. In addition to power, chemical lasers have several other advantages. These are the short wavelength of radiation, continuous operation, scalability, low power consumption, autonomy. All these factors make the industrial use of lasers promising, particularly in such areas as remote separation of materials in hazardous environments and the use in systems of special purpose. Their characteristics and principle of operation make them a unique and important object for research.
Ways to Create a Laser Environment
Introducing a large enough amount of energy into the working medium of a laser is a separate and often complex task. Since the chemical mixture already contains energy, an effective way to release it as coherent radiation should be found. To create a chemical laser, one needs a reaction that produces sufficiently high energy. It must produce elementary products (molecules, radicals, atoms) in excited energy states associated with the internal degrees of freedom (Wu et al., 2020). Moreover, the distribution of these states must be inverse. It means that there must be more molecules in at least one of the higher energy states than in one of the lower ones.
The inverse distribution during a chemical reaction can be examined using the example of a two-atom molecule. It has one vibrational, two rotational, and three translational degrees of freedom. The chemical reaction must be such that the energy released in the reaction is concentrated primarily in the excited vibrational states. The initial distribution of energy levels may be different, but in collisions with each other, the molecules are distributed very quickly (Fukuta et al., 2019). Later during collisions, energy begins to transfer from the rotational-oscillatory reservoir to the translational degrees of freedom, but the rotational energy transfers to the translational energy much faster.
As a result, the vibrational degrees of freedom remain hot, while the rotational ones cool down quickly. Therefore, most of the molecules turn out to be concentrated at low rotational levels. It is at this point that the generation of coherent radiation in a chemical laser occurs. The more efficiently energy is transferred from rotational to translational degrees of freedom, the more favorable the conditions for laser operation are (Fukuta et al., 2019). It means that it is advantageous for the translational degrees of freedom not to heat up too much. Therefore, a diluent that is chemically inert concerning the reacting substances is added to the working mixture of the chemical laser.
The distribution of molecules into energy states is nonequilibrium and can only exist for a short time. The chemically reacting mixture will heat up and will not be able to amplify electromagnetic radiation. Therefore, to realize the described mechanism of emergence of the amplifying laser medium, very fast chemical reactions, the rate of occurrence greater than the rate of establishment of thermal equilibrium in the laser medium, are required (Liu & Miller, 2021). It turns out that only reactions involving very active particles free atoms or radicals can have such rates. Hydrogen fluorination reaction turned out to be the best among them. Another way to create an active laser medium is based on energy transfer from one molecule to another particle (molecule or atom). The formation mechanism of inversely populated molecules is identical to the mechanism in a CO2 laser excited by an electric discharge.
Organization of the Chemical Process in the Laser
Chemically active radicals do not occur in the free state (the exception is the radical NO). They can be obtained by breaking a stable molecule into its constituent parts. To do this, it is necessary to expend energy equal to this molecule’s dissociation (bond breaking) energy. The radicals thus obtained will react with other molecules, in which the active medium will emerge. For example, due to energy (electric discharge, photolysis, heating), the conventional molecule AB is broken into its components: AB + Q + B, where Q is any type of energy expenditure (Wang et al., 2019). The resulting active radical or atom (let it be A) enters into a chemical reaction with molecule C, which results in an excited molecule AC, capable of radiation: A + C AC. Under appropriate conditions, it gives off the energy stored in it in the form of a laser radiation quantum AC* + nhν = AC + (n + 1) hν (Harper et al., 2019). This is the simplest kinetic scheme of a chemical laser. However, in this scheme, obtaining radicals requires energy costs.
A way out of this situation is to use a chain chemical reaction, such as a reaction involving radicals, which are renewed. Consider a mixture of two conditional molecules A2 and B2. Without initiation, the reaction in this mixture does not start. By expanding energy (for example, by illuminating the mixture with ultraviolet light), free atoms can be created (radicals): A2 + Q 2A, which start the reaction A + B2 AB + B (Yang et al., 2021). As a result, the chemically active particle B (atom or radical) is formed again, which continues the reaction: B + A2 AB + A. The initial active particle A is reduced, and the process may repeat. This process can generate laser radiation if these reactions create excited AB molecules due to the energy released.
The ultimate goal of a chemical reaction used in a chemical laser is radiation. From this point of view, what matters is not how many reaction products (AB molecules) are created during the lifetime of atom A. Still, how many excited AB molecules have time to emit a quantum of laser radiation (Yang et al., 2021). Thus, the proper length of a chain process is defined differently from the conventional chemical and laser points of view. In the first case, it is the ratio of the rate of a single reaction step to the rate of death of the active particle (chemical chain length). In the second it is to the rate of de-excitation of the emitting molecules (chemo later chain length).
The first chemical laser was based on a mixture of molecular hydrogen and chlorine: H2 + C12. This reaction is a classic representative of chain chemical processes. It turned out that in this reaction, the first link was relatively slow (Belevtsev et al., 2018). Therefore, the development of the chain as a whole is inferior in speed to the processes that deactivate excited molecules. Thus, the chemical reaction in a mixture of hydrogen and chlorine molecules is not a chain reaction from the laser point of view. Long chemo chain length reaction was later found using a mixture of hydrogen (or deuterium) and fluorine molecules.
Kinetically, the development of the chain process in this mixture is completely similar to the development of the chain in a mixture of hydrogen and chlorine: F2 + Q 2F, F + H2 (D2) HF (DF) + H (D), H (D) + F2 HF (DF) + F (Belevtsev et al., 2018). The difference is that both chain links produce vibrationally-excited HF or DF molecules. The energy released in the reaction of hydrogen with fluorine is three times the energy of hydrogen with chlorine. Finally, the most important circumstance: the rate of both links of the reaction of hydrogen with fluorine is higher than the rate of relaxation processes.
Continuous Chemical Lasers
In order to get generation in a chemical laser for a long time, people need to change reagents in the reactor quickly enough. Additionally, they can produce chemically active centers continuously for the entire duration of the laser. A long-duration chemical laser consists of a source of chemically active centers. A system for mixing free atoms or radicals with another component, the reaction with which produces excited molecules. Another part is the reactor, where the active laser medium is created, and the optical resonator (Guan et al., 2018). In addition, a fast feed of initial reagents and a pumping of spent reagents are required.
This general scheme is practically realized in various types of continuous chemical lasers (DF-CO2)-laser. This laser is based on energy transfer from vibrationally excited DF fragments to CO2 molecules in a D2 + F2 + CO2 mixture. The reaction is initiated by a radical: NO + F2 NOF + F, followed by a chain reaction of deuterium with fluorine (Zhao et al., 2018). The resulting DF particles quickly transfer energy to CO2 molecules, which serve as the emitter. A schematic of such a laser is presented in Figure 1. If carbon dioxide molecules are not introduced into the reacting mixture, it is also possible to achieve generation by emitting DF molecules in the same laser system. However, experiments have shown that DF (or HF-) lasers operating according to this scheme are markedly inferior to (DF-CO2)-laser. The point is that excited molecules live longer than DF or HF molecules.
HF(DF-)-laser with thermal initiation of reaction; in molecular fluorine, hydrogen and helium are introduced into the combustion chamber. Fluorine is in large excess relative to hydrogen. The normal equilibrium combustion of hydrogen in fluorine occurs in the combustion chamber, releasing enough heat to dissociate the excess molecular fluorine. The reaction in a highly heated mixture also cannot be used to create an active medium: the mixture must be cooled. The mixing of these two streams results in a working mixture, the reaction in which F + D2 DF+ D leads to the formation of vibrationally-excited deuterium fluoride molecules that generate laser radiation.
The key problem here is the mixing process; it must be fast. Therefore, the nozzle block is an array of small nozzles that divide the flow into jets of small cross-sections – about 1-2 mm. Furthermore, in the reaction of atomic fluorine with deuterium, a considerable amount of energy is released. Part of this energy (about 15%) is carried away by laser radiation (Avdeev & Katorgin, 2020). The rest eventually heats the flux. It is known that heating can greatly slow down the supersonic jet and even suppress the supersonic flow process. And this completely upsets the harmonic conditions necessary for laser radiation generation. Such deactivation is particularly active if vibrationally-excited molecules of the same sort are emitted.
This is why deuterium is introduced into the laser zone if the combustion chamber uses hydrogen as fuel. The oxygen-iodine laser uses singlet oxygen energy. Their rate is low compared to the hydrogen fluorination reaction. However, singlet oxygen lives much longer than a vibrationally excited hydrogen fluoride molecule. Each singlet oxygen molecule carries an energy reserve of about 1 eV. This energy cannot be used directly, so the flux of singlet oxygen is mixed with the flux of iodine molecules I2 (Zhang et al., 2017). Due to the energy of singlet oxygen, the iodine molecule breaks into atoms. The resulting atoms of iodine, when colliding with singlet oxygen, go to the excited state. The excited atomic iodine forms the active medium of the laser.
Figure 1. Schematic of the chemical (DF-CO2)-laser of continuous operation based on the chain reaction of deuterium fluorination:
- atomic fluorine generation chambers,
- mixing chamber,
- reactor zone,
- windows for radiation output into the resonator,
- blank resonator mirror,
- translucent mirror of the resonator.
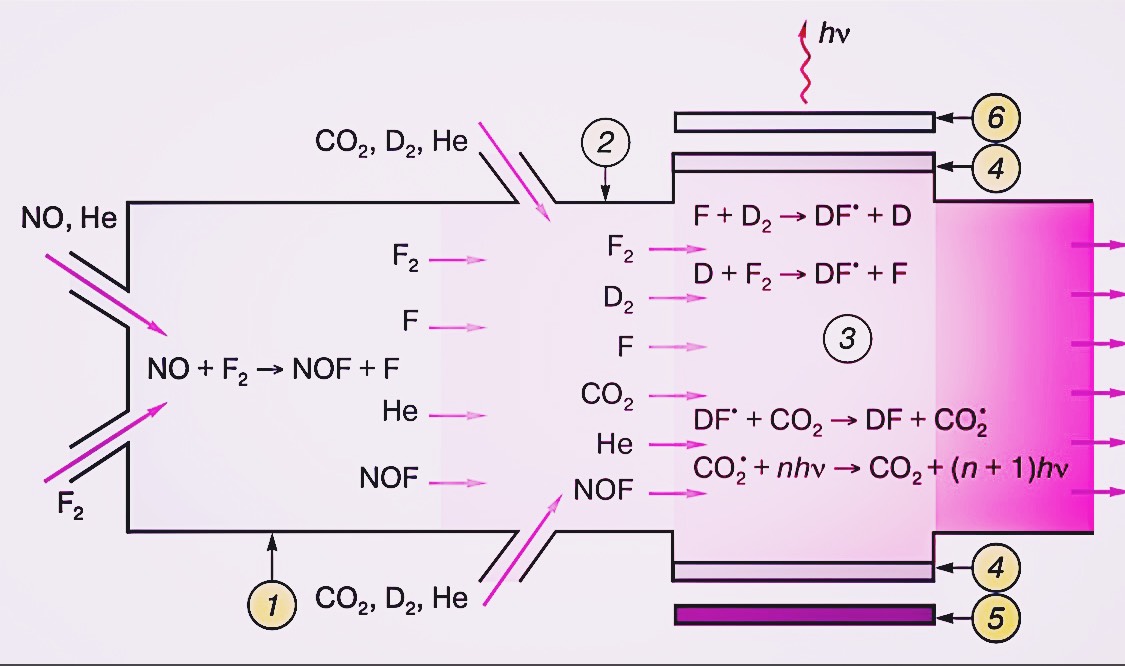
Pulsed Chemical Lasers
Like pulsed lasers of any other type, these lasers are designed to produce a short, powerful pulse of coherent radiation. A pulsed chemical laser consists of the following basic components. That is the reactor, where the fast-chemical reaction, in which the active medium is produced. Additionally, it includes an optical resonator, a system of reaction initiation, preparation, and injection of the reagent mixture into the reactor, and removal of the waste products (Jeong et al., 2017). Figure 2 presents a typical diagram of such a laser. The chemical components shown in the diagram can be replaced with others. Reaction initiation in the given scheme is performed by the ultraviolet radiation of a pulsed lamp.
The reaction can also be initiated by a high-energy flow of electrons or an electric current, which flows through a mixture of chemical reagents in the reactor. As already noted, to obtain an effective chemical laser with low reaction initiation costs, it is essential to apply chain chemical reactions with long chemo later connection length. Compounds of H2 + F2, D2 + F2, D2 + F2 + CO2 have this property. The mixtures used in practice are usually are more complicated. Thus, oxygen O2 is added to these three mixes to stabilize them within a few percent of the total mixture volume (Duta et al., 2017). Often an inert buffer gas is added to the compound to prevent the mixture from heating up too much and accelerate the relaxation processes that help create the active laser medium. When an electron flux initiates a reaction, molecules with large weight are added to the compound to increase the concentration of chemically active centers.
The properties of pulsed chemical lasers are record-breaking for gas lasers. However, the main disadvantage of a pulsed chemical laser compared to a continuous laser is that the pulsed laser has not yet been made purely chemical. Nevertheless, there is an idea of creating such a laser. It consists in making the radiation generated by the laser not only be the end product of the method. Although also cooperate in developing the chemical reaction itself, breaking the molecules contained in the mixture into chemically active radicals. (Duan et al., 2017). Then the source of the initiating energy will ultimately also be a chemical reaction, and the pulsed laser will be purely chemical. Theoretical studies show that this scheme is also fundamentally feasible, but it has not yet been realized in practice.
Figure 2. Schematic diagram of a pulsed chemical laser:
- mixer,
- refrigerator,
- reactor,
- blank mirror,
- partially transparent mirror for the radiation output,
- window holders and chambers for protection of windows from corrosion by reaction products,
- flame arrestor,
- chemical absorber,
- flash lamp.
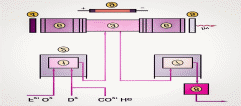
The Importance of Chemical Laser
It is worth studying chemical lasers from a theoretical rather than a practical point of view. Chemical reactions accompanied by emission of infrared, visible, and even ultraviolet radiation were known in the pre-laser era. The creation of chemical lasers showed that this radiation could be coherent. Different kinds of energy have different ‘quality’; in science, there is a degree of quality of energy – entropy. The lower the entropy, the higher the quality of energy. In a state of thermal equilibrium, entropy is maximum for that amount of energy, so heat is the lowest quality energy. Over hundreds of years of using chemical energy, its view as a source of heat has been shaped (Trusovas et al., 2016). Research stimulated by the development of chemical lasers convinces us that a wide class of chemical reactions is a source of low entropy energy. From a practical point of view, one should note that the most powerful of the continuous chemical lasers that engineers have created is the chemical HF laser with thermal initiation of the reaction. Specialized technological systems can be made based on massive chemical lasers.
Chemical lasers with their energy autonomy and high specific energy can be used in laser space technology. No matter how much nuclear power engineering is criticized nowadays, no alternatives have been found for it in the power engineering of the future yet. One option for using nuclear (thermonuclear) energy is to build power plants away from dense habitats. In these areas, nuclear reactor energy is converted into chemical energy by decomposing water into hydrogen and oxygen (Trusovas et al., 2016). Hydrogen as a combustible gas is delivered to the consumer via a gas pipeline. Since in such a project, the main type of fuel extracted with nuclear energy is hydrogen, creating a chemical laser based on the hydrogen oxidation reaction looks very attractive. Apparently, along with the creation of a purely pulsed chemical laser, this is one of the most interesting assignments in the field of chemicals.
Conclusion
A chemical laser is a tool that receives energy from a chemical reaction. They can achieve continuous-wave outputs of up to megawatts. The chemical lasers described above are the best performing and most advanced chemical lasers in terms of engineering. Although there are other systems of chemical lasers based on the reaction of carbon disulfide in which CO molecules are the emitter. It attracts attention in terms of the function of chemical reaction kinetics.
References
Avdeev, A. V. E., & Katorgin, B. I. (2020). Investigation of the process of mixing reagents in the laser chamber of active medium generators of continuous-wave chemical lasers with promising nozzle arrays. Quantum Electronics, 50(10), 917.
Belevtsev, A. A., Firsov, K. N., Kazantsev, S. Y., Kononov, I. G., & Podlesnykh, S. V. (2018). Self-sustained volume discharge in mixtures of SF6 with hydrocarbons, hydrogen and deuterium for non-chain HF (DF) lasers. Journal of Physics D: Applied Physics, 51(38), 384003.
Duan, B., Zhang, H., Hua, Z., Wu, L., Bao, Z., Guo, N., & Shen, R. (2021). Impulse and electric charge characteristics of chemical propellant under pulsed laser irradiation. Vacuum, 192, 110419.
Duta, L., Mihailescu, N., Popescu, A. C., Luculescu, C. R., Mihailescu, I. N., Çetin, G., & Stan, G. E. (2017). Comparative physical, chemical and biological assessment of simple and titanium-doped ovine dentine-derived hydroxyapatite coatings fabricated by pulsed laser deposition.Applied Surface Science, 413, 129-139.
Fukuta, S., Nomura, M., Ikeda, T., & Wakabayashi, K. (2019). Processing characteristics of laser micro incising and possibility of its high-speed processing. European Journal of Wood and Wood Products, 77(2), 249-255.
Guan, X., Zhan, L., Zhu, Z., Xu, B., Xu, H., Cai, Z., & Xu, J. (2018). Continuous-wave and chemical vapor deposition graphene-based passively Q-switched Er: Y 2 O 3 ceramic lasers at 2.7 μm.Applied optics, 57(3), 371-376.
Harper, K. C., Moschetta, E. G., Bordawekar, S. V., & Wittenberger, S. J. (2019). A laser driven flow chemistry platform for scaling photochemical reactions with visible light. ACS central science, 5(1), 109-115.
Jeong, K., Lee, J., Byun, I., Seong, M. J., Park, J., Nam, H. S., & Lee, J. (2017). Pulsed laser chemical vapor deposition of a mixture of W, WO2, and WO3 from W (CO) 6 at atmospheric pressure. Thin Solid Films, 626, 145-153.
Liu, J., & Miller, T. A. (2021). Laser-Induced Fluorescence Spectroscopy of Large Secondary Alkoxy Radicals: Part I. Spectral Overviews and Vibronic Analysis. The Journal of Physical Chemistry A, 125(7), 1391-1401.
Trusovas, R., Račiukaitis, G., Niaura, G., Barkauskas, J., Valušis, G., & Pauliukaite, R. (2016). Recent advances in laser utilization in the chemical modification of graphene oxide and its applications.Advanced Optical Materials, 4(1), 37-65.
Wang, D., Kelkar, H., Martin-Cano, D., Rattenbacher, D., Shkarin, A., Utikal, T., & Sandoghdar, V. (2019). Turning a molecule into a coherent two-level quantum system. Nature Physics, 15(5), 483-489.
Wu, J., Zhao, J., Qiao, H., Hu, X., & Yang, Y. (2020). The new technologies developed from laser shock processing. Materials, 13(6), 1-20.
Yang, N. N., Zhang, J. L., Zhao, Y. L., LI, G. F., & Wen, X. Q. (2021). Discharge mode analysis of pulsed chemical HF laser system. Nuclear Fusion and Plasma Physics, 41(3), 284-288. doi: 10.16568/j.0254-6086.202103016
Zhang, P., von Freyberg, A., & Fischer, A. (2017). Closed-loop quality control system for laser chemical machining in metal micro-production. The International Journal of Advanced Manufacturing Technology, 93(9), 3693-3703.
Zhao, L., Shang, Q., Gao, Y., Shi, J., Liu, Z., Chen, J., & Zhang, Q. (2018). High-temperature continuous-wave pumped lasing from large-area monolayer semiconductors grown by chemical vapor deposition. ACS nano, 12(9), 9390-9396.