Introduction
A Radionuclide is a radioactive isotope of an element having extranuclear energy, which makes it unstable. All elements can have radionuclides and an example is a hydrogen that has a radionuclide, tritium. Radionuclides of heavy-metal elements such as uranium, thorium, cesium, have longer half-lives. These are produced in nuclear reactors, particle accelerators, and cyclotrons which are used for radio imaging and medical scanning devices, by the oil and gas industry, and they also occur in nature. Large and continuous exposure to nuclear radionuclides is harmful to live organisms. Generally, areas around nuclear sites are regulated for radionuclides in drinking water, soil, and plants [1].
While a nuclear accident would immediately render the accident area unsafe, the danger is present from gradual leaching of radionuclides from water used in the nuclear power plants, from contamination, and other pathways, into the soil and water system. Other pathways are airborne particulate matter that is emitted from nuclear power plant stacks. These radionuclides are absorbed by the soil, they are washed down due to precipitation and rain, and they enter underground water streams. Plants and vegetables that consume these waters also imbibe the radionuclides, which enter the plant system and they are deposited in the leaves, stems, flowers, and fruits. The soil-plant pathway allows for long-lived radionuclides to enter into fruits and flowers.
The amount of radionuclides depends on the concentration in soil, leachability of radionuclide and the solubility, and the sorption capacity of soil particles [2]. The safe or permissible level of radionuclides in soil, water, food, and various parts of the body is not clear. The United Nations Scientific Committee on the Effects of Atomic Radiation (UNSCEAR) provides a list of tables giving the level of exposure to different levels of natural radiation in different regions and countries. The level of exposure from cosmic radiation is 0.3-1.0 mSv (millisievert), for external terrestrial radiation, it is 0.3-0.6 mSv, for inhalation exposure it is 0.2-1.0 mSv, and for total ingestion it is 0.2-0.8 mSv. Exposure of 100 mSv/ year can lead to cancer [3]. This research studies the kinetics of leaching of long-lived radionuclides from UAE urban soil samples using radioanalytical techniques and plasma mass spectrometry.
Radionuclides Types
As per their origin, radionuclides are present in four main classes in the environment. These are cosmogenic, primordial, anthropogenic, and natural decay series [4]. Primordial radionuclides were developed when the earth was formed and they have very long half-lives. Some of these are 40K with a half-life of 1.248 x 109 years, 232Th with a half-life of 1.405 x 1010 years, and 238U with a half-life of 4.468 x 109 years. Natural decay series are formed by the decay of primordial radioactive isotopes and some examples are 232Th, 235U, and 238U. Cosmogenic radionuclides are formed due to cosmic radiation and some examples are 14C and 10Be. The process of decay is linked to the emission of subatomic particles and the decay of these daughters creates about 80% of radiation. Humans create short half-life anthropogenic radionuclides through nuclear power plants and weapons testing, nuclear fuel processing, dumping of fissile material and equipment, industrial activity related to oil and natural gas extraction, and other such activities. Some examples are 131I and 137C [5].
These radionuclides can enter the body through ingestion from water and food and by inhalation. Among these, radionuclides of Uranium such as 235U are of particular concern, since the use of nuclear power for energy generation is increasing. Natural uranium occurs in the lithosphere and mineral rocks of uranium ore. While uranium ore is not very hazardous, the chemical process of leaching to recover the ore is very hazardous. UAE faces the danger of anthropogenic radiation [6].
Soil Radiation in UAE
The UAE has constructed four nuclear power plants at Barakah and the plants will be operational by 2020. However, UAE has a huge oil and gas industry, and this industry has emitted large amounts of radionuclides over the past few decades. Also, there is radiation from natural sources. The permissible level of radiation varies from country to country. The European Union has set a figure of six millisieverts a year as the maximum allowed exposure [7]. While occasional exposure to radionuclides in small quantities is not harmful, radiation can create health hazards when people consume contaminated food items like dates, which could be exposed to high levels of radionuclides [8].
Published research on assessing the levels of radionuclide concentration in UAE is scant. A few researchers [7] [9] [10] have presented research associated with gross β and α from radionuclides in soil and water. The researchers collected samples of soil and water from different locations and tested them using standard lab equipment. However, research on the concentration of radionuclides and leaching mechanisms in soils at different pH values is not available. This research aims to fill this gap.
Aims, Objectives, Research Questions, and Problem Statement
The research aims to study the pattern and the kinetics of leaching of long-lived radionuclides from UAE urban soil samples using radioanalytical techniques and plasma mass spectrometry.
The objectives of the research are:
- To identify the extent and possible impact of radionuclide/ metal leaching in soil on the environment.
- To generate data of the leaching process and the kinetics of leaching and to add to the scientific knowledge in those areas about UAE.
- To assess the effect of pH on metal leaching to find possible solutions to control/ curtail the harmful effects of leaching.
- To contribute to the body of knowledge about the Naturally Occurring Radionuclides (NORMS) and Technically Enhanced NORMS (TNORMS) data for UAE.
Research questions proposed are:
- What are the current levels of Naturally Occurring Radionuclides (NORMS) and Technically Enhanced NORMS (TNORMS) in UAE soil?
- What type of data will be generated by the leaching process and the kinetics of leaching studies and what scientific knowledge can be developed on radionuclides/ metal leaching from the soil?
- What indicators can be developed to assess the extent and impact of radionuclide/ metal leaching on the environment?
- How can data on the effect of pH on metal leaching be used to find possible solutions to control/ curtail the harmful effects of leaching?
The problem statement is: “How can the leaching studies and the radiation measurements indicate the level of long-lived radionuclides that can migrate from the soil to the borehole water and agricultural run-off in UAE?”
Project Timelines
The Gantt chart given in Fig 1.1 illustrates the proposed project line, milestones, and time required to complete them.
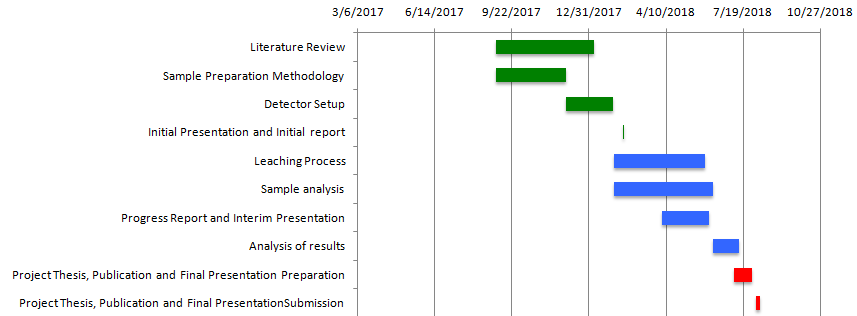
Literature Review
As indicated in section 1.3, research on assessing the radionuclide levels in UAE soils is scant. A review of literature on UAE and in other regions, along with the kinetics of leaching is presented in this section.
Leaching and Transfer of Radionuclides Through Soil-Root Pathway
The kinetics of nutrients and other mineral matter by soil is important [11]. The author points out that colloids, or clay mineral, organic matter, and humus are important channels since they are permeable, they have a higher surface area, and they have higher chemical activity. Silt, sand, pebbles, and rocks are hard, not permeable, and serve as skeletons to hold colloids in place. Clay has molecules with negatively charged oxygen atoms at the top and lower surface. These clay particles attract the positive ions of H+, Na+, Ca2+, and other elements and compounds. Cation exchange theory is important to understand the replacement order, where cations replace other ions. At low and ordinary temperatures, replacement speed for univalent cations at constant valence shows an increase when the size of the hydrated ion decreases. When the same ambient conditions exist, the exchange frequency rises with more valence of exchange cations, so Na+< Ca2+< Al3+<Th4+, and other exchanges occur.
It is interesting to see how radionuclides are adsorbed in the soil. Cesium is a positively charged ion and it is adsorbed by soil colloids, and uranium radionuclide is also positively charged and adsorbed. However, further pathways and entry into the plant depend on the pH value and organic content of the soil. The model for migration and movement of radionuclides in the soil is illustrated in Fig 2.1.
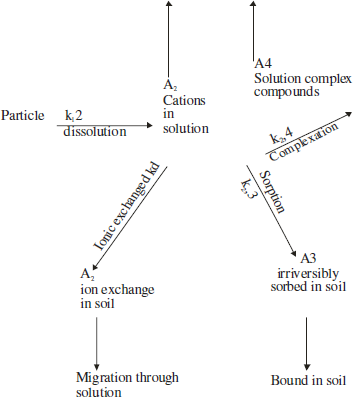
The Soil-root pathway is important in the transfer of radionuclide into fruits since this pathway is available for all plants growing in an area with increased nuclear radiation. Particulate radionuclides, deposited on the soil are usually not easily soluble in water. Rather, they are leached gradually through a weathering process. The equation to describe the flux of radionuclides leached into the soil is given as follows [12] [13] [14]:
dQ/dt = – kwQ-kpQ
Where:
- kw is the leaching rate from weathering
- kp is the physical decay rate
- Q is the number of radionuclides deposited in the soil, calculated as unit/ L2
When radionuclides are leached into the soil are sorbed by the particles, equilibrium of distribution is defined between the soil solution and soil particles, and this is given by the expression:
Cp – Kd Cs
Where:
- Kd is the distribution coefficient L3/ M
- Cp is radionuclide concentration in soil solution, unit/ M
- Cs is the radionuclide concentration in the soil solution, unit/ L3
The total radioactivity/ unit area of the ground Ctotal is given as:
Ctotal = D(m +BdKd) Cs
Where:
- D is the depth of the root zone, L
- m is the volumetric content of moisture in the soil
- Bd is the bulk density of soil M/ L3
Assuming that absorption by plants is proportional to the plant weight and size and soil solution, and then the relation is.
d (p x fg)/ dt = kb x fg x Cs – kp (P x gf)
Where:
- P is the radionuclide concentration in the plant, Unit/ M
- fg is the function of plant growth (M/ L2 at time t)
- kb is the uptake rate constant of the plant (L3/ M/ T)
Research on Leaching of Radionuclides in Soils
Rihs et al examined the chemical kinetics and dynamics in soils of France for the Uranium and Thorium series [16]. Gamma spectrometer was used to find the levels of 238U, 230Th, 226Ra, 232Th, 228Ra, and 228Th in water seepage, solid particles, and mature leaves of beech trees. The use of gamma spectrometer helped to understand the preferential Th leaching over that of Ra and the disequilibria was noted. It is seen that Ra and Th transfer follow the protocols of acido-complexolysis weathering methods. The continuous leaching method was used to destabilize 226Ra and 230Th and it was measured for soil samples collected at different depths and helped to date the processes in the soils. It was seen that there is preferential leaching of Th from shallow regions due to the higher presence of organic colloids that started about 18 years back. Fig 2.2 illustrates the products and processes of petrochemical plants that cause radiation.
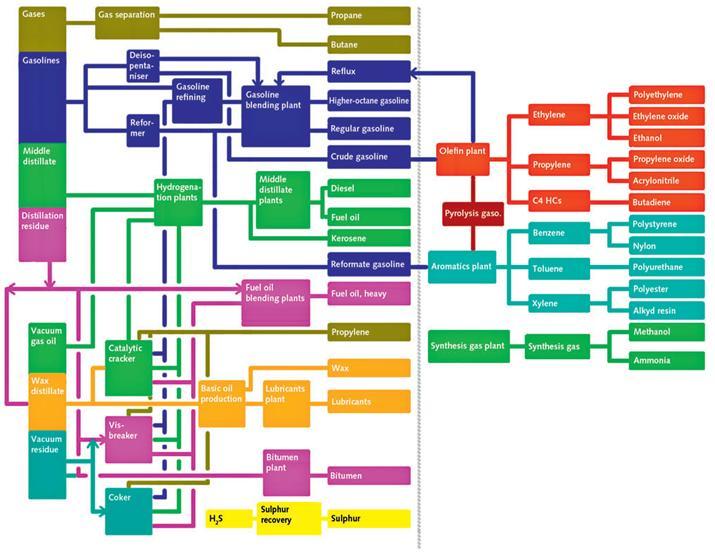
High levels of radionuclides from activities such as oil and gas, phosphate mining, commercial, and shipping activities tend to increase the level of radionuclides. The Middle East has not been well mapped to understand the distribution and presence of radioactive concentration. Ababneh et al. studied the concentration of radioactivity concentrations in the Gulf of Aqaba, Jordan [18]. The study was to establish baseline data and understand the impact on the marine environment. The researchers studied the radioactive levels of gamma-emitting radionuclides in beach and core sediments of the Gulf of Aqaba regions. Five areas were identified and core sediments were collected from water depths of 5, 15, and 35 meters.
Beach sediments were collected from pre-marked locations. Cores were collected by scuba divers and placed in Perspex corers and taken to the lab and frozen. The frozen cores were sliced into three layers, air-dried and processed. The samples were then tested using gamma spectrometers. The samples were oven-dried, crushed, homogenized, sieved, and then tested. The findings indicate that concentrations of 238U, 235U, and 226Ra for seafloor and beach sediments from the areas of phosphate-rich zones, had levels that were twice higher than the values from other locations. The level of 238U concentration was in the range of 57-667 Bq Kg-1. The results also indicate that there is less variation of radioactivity concentration in the core length of 15 cms. It was seen the mean values of radium equivalent Raeq, Hex the external hazard index and the absorbed dose rate were 628 Bq Kg-1, 283nGy H-1, and 614 mSv y-1. These values are very much greater than the allowed values and pose significant health risks.
Rahman and Solodov researched to develop a radiation baseline in the UAE before the first nuclear reactor became operational [19]. They studied gamma-emitting radionuclide concentration in palm dates, from trees growing in the UAE region. Samples from 10 types of palm dates were obtained for analysis. They examined naturally present radionuclides such as 232Th, 238U, 40K, 137Cs, and others. The samples were prepared by crushing and oven drying to remove the water content, and then tested using a gamma spectrometer. The spectrometer measurement showed concentrations of 0.61-0.89 Bq Kg-1 for 238U, 0.10-0.23 Bq Kg-1 for 232Th, and 191-362 Bq Kg-1 form 40K. Wet-condition concentrations were also measured and they showed values in the same range, though the variation was less.
Some areas of the Middle East such as Kuwait have seen research on radionuclides in sand and soil. This area had seen intense fighting in 1991 during the Gulf War and hundreds of oil wells were set on fire or damaged, causing huge oil spills that formed large surface deposits. The war also saw the use of more than 800 tons of depleted uranium shells for missiles [20]. Therefore, it was essential to see the concentration levels of radioactive material in the region. Jallad carried out a study to find radioactive characterization in North Kuwait [21]. For the study, 16 sites were selected and sand samples were collected from a depth of 5 cms, and sealed in a plastic bag, before sending them to a radioactive analysis lab in the US.
The samples were dried in an oven at 103 0C, pulverized, and homogenized. Sealed containers were used for preparing the samples. Samples were collected from these processed particles and checked with a gamma spectrometer. Samples were checked for 238U, 226Ra, 40K, 232Th, 137 Cs, and 131I. Spectra of the samples were obtained and the gamma photo peaks were measured. Misidentification was removed for 236Ra that emits a gamma-ray of 186 Kilo Electron Volt (KeV), similar to the emission of 235U. Radionuclide concentration and Minimum Detectable Concentration (MDC) was measured using the spectrometer. Findings indicate that 238U level was 11Bq/kg, 232 Th was 30 Bq/kg, 226 Rawas 12 Bq/kg, 40K was 397 Bq/kg, 137Cs was 2.0 Bq/kg, and 131Istood at 19.0 Bq/kg. The conclusions formed are that the concentration levels were found to be lower than the international levels. The assessment is that sand is impermeable and leaching is very low. Hence, the levels were below the expected concentrations. Fig 2.3 illustrates the Thorium decay lifecycle.
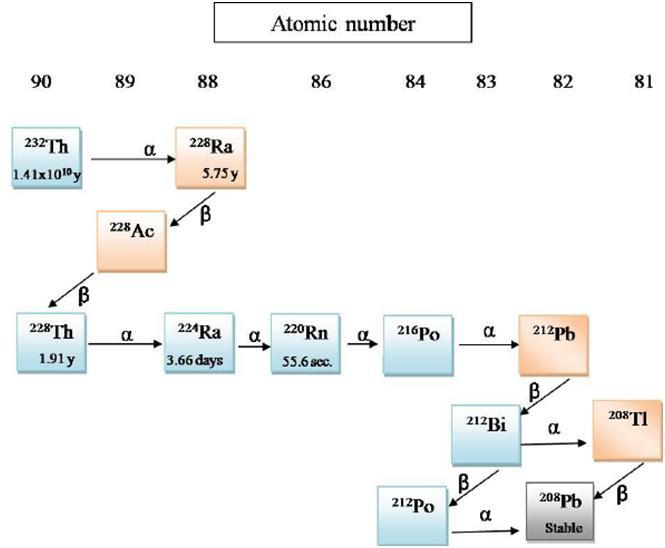
pH and other natural conditions such as sorption capacity, redox potential (Eh) have a significant impact on radionuclide concentration in the soil. Koch-Steindl and Pröhl present a discussion and analysis to understand the effect of pH on radionuclides in soils [22]. The authors argue that the soil system is very complex and the behavior of radionuclides is based on the elemental properties, minerals, and colloids in the soil. One of the main factors that regulate the kinetics of leaching is the redox potential, which is linked to the pH value of the soil. To assess the radionuclide behavior, Eh-pH stability diagrams are used. Redox processes happen when water molecules are present. The stability of water depends on the decomposition of water into oxygen, while the lower limit depends on the decomposition of hydrogen. The equations formed are.
1/2 H2O(l) →1/2 O2(g)+2 H+(aq)+2e–2 H+(aq)+2e–→H2(g)
The redox potential and the pH value are therefore negatively related. When the Eh decreases, the pH increases, and vice versa. In the above equations, the first reaction creates protons and decreases pH, and the second equation uses protons to increase pH. For optimum agriculture use, the pH value is kept near neutral. Hence, leaching of radionuclides is steady at the neutral pH values, it is higher in sandy soils with low pH value, since sandy soils have larger concentrates of lime and carbonates, and leaching is slower in higher pH soils that have more clay. However, the absence of water and moisture in desert areas, reduce the leaching effects [23].
Gil-García et al. present research to support the inverse relation of radionuclide leaching rate and pH level [24]. The researchers collected soil samples from 20 quarries in Nigeria to measure the presence of radionuclides and the relation with particle size, organic matter, carbon, and pH values. The samples were studied to find 40K, 228U, and 232Th presence. The samples were gathered from various depths, air dried, pulverized, and passed through a sieve of 2 mm mesh size. pH was measured by mixing 2 grams of the samples with 20 ml of distilled water and left for 10 minutes to settle. The mixture was then stirred and allowed to stabilize for 30 minutes. A pH meter was used to measure the pH and the results analyzed. The remaining samples were then tested to find the concentration of radionuclides. The concentration of pH values and radionuclides varied between the quarries from which they were selected. However, it was seen that with an increase in radionuclide levels, pH values fell to below six.
Kd value is the ratio of the concentration of solid phase elements to the equilibrium concentration in the liquid phase [25]. When the Kd value is large, then the amount of element adsorbed to solid particles is less, the mobility decreases because of less water content. Kd values are used to indicate the retention of radionuclides in soils. The values are usually measured for a specific location [26]. The relation between Kd values, adsorption, and leaching is not linear and it depends on some parameters such as temperature, pH value, soil content and colloid content, and others. Sohlenius et al. researched to understand the mobility of different elements that affect the leaching and movement of radionuclides [27]. The objective was to assess if chemical properties of soils can be used for cultivation in the future when large amounts of radionuclides and other metallic contaminations would be mixed with farm soil due to increased industrial activity. The study examined the solid/ liquid partition coefficient, Kd values that can impact the mobility of elements.
The researchers gathered soil samples from the Forsmark region of Sweden. GIS maps were used to identify specific areas with wide differences in soil chemistry and structure. Samples were taken from different depths of 20-25 cm and 50-55 cm. Pore water and solid-phase samples were collected and they were dried for one week. The soil samples were digested with aqua regia and analyzed using inductively coupled plasma sector field mass spectrometry (ICP-SFMS). Other parameters such as pH and mineral content with constituents of different minerals were also measured. The effects of these parameters and depth at which samples were taken on Kd values were measured. The findings indicate that concentrations of radionuclides of Cesium, Nickel, Uranium, 226Radium increased when the pH values were below 5.5, and the values decreased when the pH values were in the 8-8.5 range. These findings are important since the soil in UAE, in the desert region, is considered as acidic, leading to higher levels of radionuclides [28].
Methodology
In previous research, soil samples were collected from urban areas of the UAE. These will be processed and leached in this research. The earlier samples were taken from different depths and different terrains. The samples were collected and stored in sealed containers, as per the process recommended by Khalifa University [29]. These samples will be now be leached at the university laboratory. Modern gamma-ray instrumentation and Inductively Coupled Plasma Mass Spectrometry (ICP-MS) will be used to study the leachate. Samples will be segregated as per the depth at which they were collected and the soil pH value. A previous study done in UAE will serve as the model for the leaching process and kinetic study. Toxic level estimation of arsenic, cadmium, lead, and mercury will be carried out by ICP-MS equipment. Fig 3.1 illustrates the schematic layout of ICP-MS.
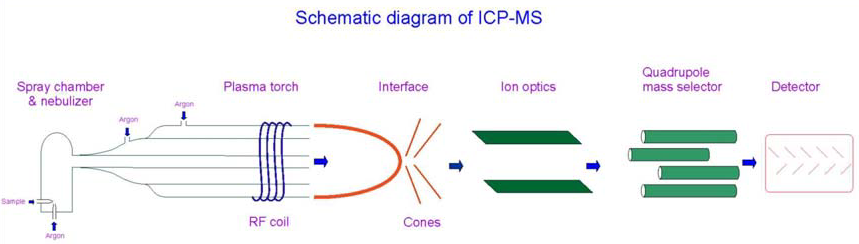
Sample Preparation
Samples were collected in previous research from different locations in the UAE, and these will be processed and leached in this research. The Google Map in Fig 3.2was first prepared to cover the area under study. Location coordinates of each sampling area were noted down so that it will be easy to pinpoint the exact location from which a sample was taken. A hand-operated sampling drill was used to dig into the earth and five grams of each sample was collected in polypropylene containers [28]. Fig 3.2 illustrates the Google map of the locations.
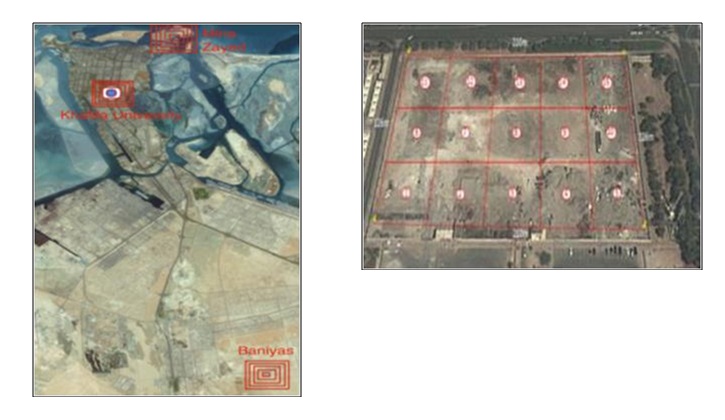
The following radionuclides were found in the previous research228Ac, 212Pb, 212Bi, 208Ti, 226Ra, 214Pb, 214Bi, and 40K (Al-Ali 46). In this research, the samples will be leached for 12-16 weeks. De-ionized water of three pH levels – acidic, neutral, and basic, will be used to evaluate the effect of pH on metal leaching from soil samples [28]. Leachate samples will be collected every week and analyzed with the ICP-MS. To avoid contamination, special polypropylene tubes will be acid washed to get rid of all surface contaminations before the leaching process. The samples will be stored at room temperature and mixed regularly using an orbital shaker.
ICP-MS – Instrument Details
Inductively Coupled Plasma Mass Spectrometry (ICP-MS): ICP-MS is an instrument which will help to identify and quantify different isotopes of elements in the samples. This study uses the Perkin Elmer DRC-e model. The instrument has wide detection limits that extend to the ppt level for certain elements, with higher throughput for fast sequential scanning. The instrument detects multiple elements in a single scan. Additional features such as collision/ reaction cell to minimize matrix interferences extends the capabilities of the device [30].
Plasma at atmospheric pressure holds the ions and strikes the exterior cone. Ions enter the high vacuum region through the cone orifices where the mean collimating system, quadruple ion selection systems, shadow stops, and detector are placed. The orifice is then sealed by the plasma, blocking external air from entering. Pumps provide a high vacuum inside the chamber. Four rods of gold plates molybdenum rods are used to apply voltage to allow a single ion to pass through the detector. The high-temperature plasma is maintained by the RF source through an inductance coil with a mass spectrometer. Argon gas passes through the plasma torch and an initial spark removes the argon atoms to form the ions. These ions are trapped in the oscillating magnetic fields formed by the inductance coil, setting a chain reaction of collisions to maintain a stream of Ar ion. The detector identifies individual radionuclides and the results are printed as reports [31]. Fig 3.3 is the photograph of the ICP-MS instrument in our lab.
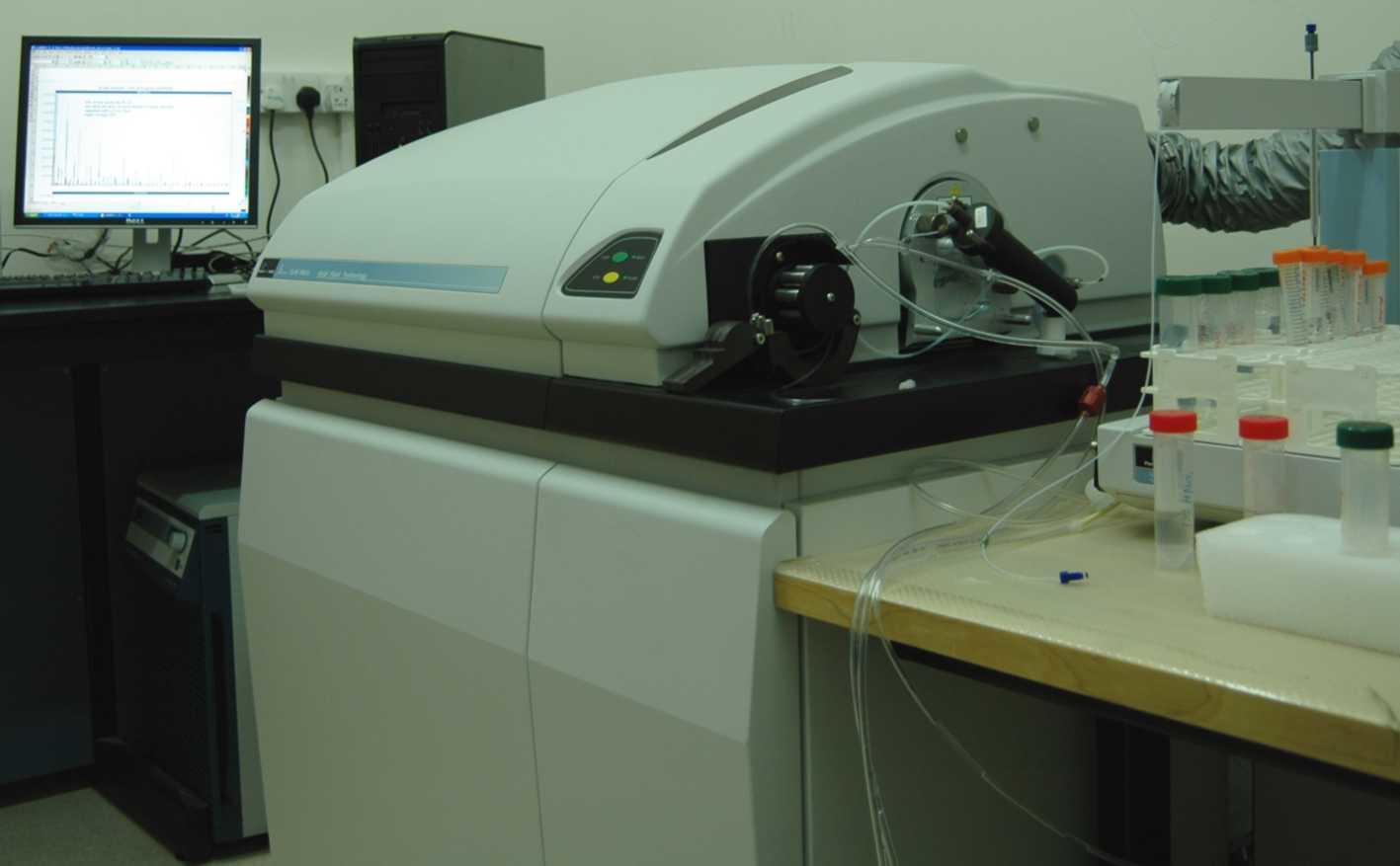
Liquid Scintillation Analyzer
This section presents a review of the liquid scintillation analyzer. Lab procedures will be submitted later. The Tri-Carb® 3110TR instrument is a liquid scintillation analyzer used to detect alpha, beta, gamma radioactivity, and luminescence in samples. The instrument operates with processes such as beta particle and photon production and reduction. The instrument has several features that provide for the lowest backgrounds with replay sample recall and reprocessing, which reduces lost data. It is used extensively for advanced analysis and studies for 1, 2, and 3 radionuclides. The main components of the system are the scintillation detector, pulse analyzer, sample changer, spectrum analyzer, system and display, data output and recorders, and the microprocessor [32].
The automatic sample changer allows for the movement of the sample from the detector. Samples are placed in cassettes to a load station and into the detector on paired fabric belts to carry the samples in and out of the detector. The most important part of the instrument is the spectrum analyzer. The coincident summed pulses from the detectors are stored in the analyzer. Linear amplification provides stability and data are written in the control until the termination is reached. Preset conditions are defined by systems and these are 3H, 14C, 32P, and 125I in single and double label measurements. The user can determine regions by specifying the energy limits of radionuclides or by empirically setting values. The full spectrum of radionuclides is gathered during sample measurement without loss of counts and time. A spectral index of the sample is calculated and this is related to the quenching of the sample. The instrument stores spectrum data in 4000 linear channels. The data can be subjected to visual presentation analysis, region optimization, vector qualitative analysis, pattern recognition, time-resolved spectrum analysis, spectrum unfolding, direct DPM calculation, reverse spectrum transformation, and efficiency tracing [33].
Other important functions are data reduction and data output. The instrument allows automatic calculations of Counts per Minute (cpm), auto background subtraction, averaging, low count rate rejection, floating decimal point readout, decay computations, normalization, single and dual half-life correction, and direct DPM and efficiency tracing. Users can define protocols and add custom reduction software to the system. Data output is available in spreadsheets and users can specify the data output format. Formulas can be applied to different cells to customize outputs. Luminescence detection and correction is an important part of the instrument and it allows the spectral stripping of chemiluminescence and photoluminescence events as the percent of total detected counts [34]. Fig 3.4 is the photograph of the liquid scintillation analyzer instrument in our lab.
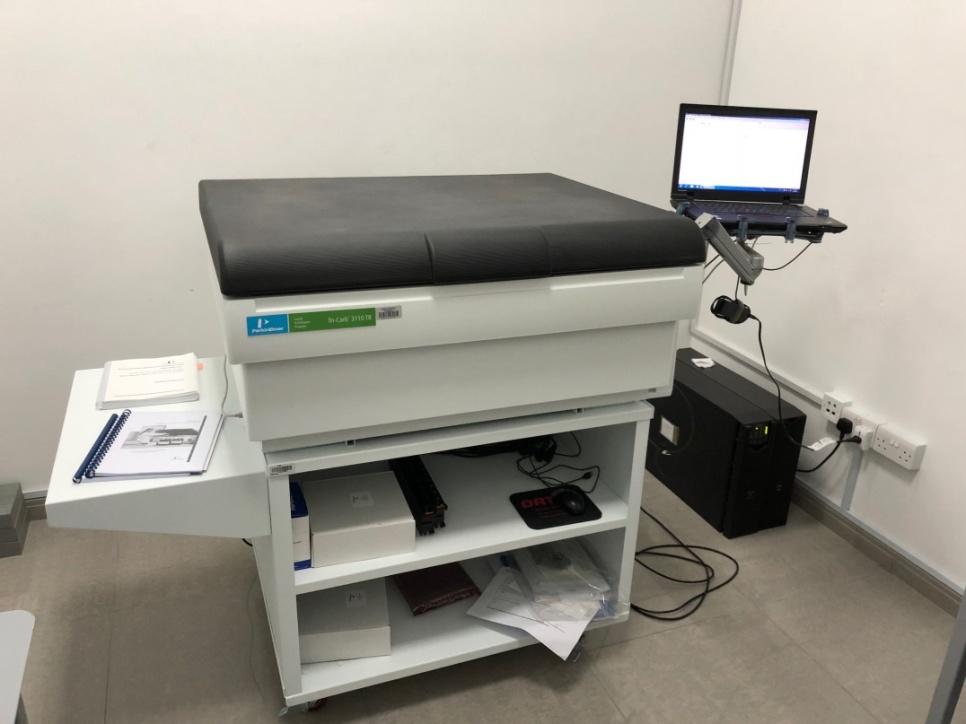
Another view of the instrument is shown in Fig 3.5.
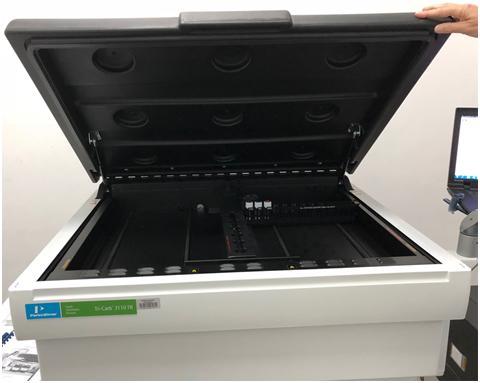
A closer view of the instrument is shown in Fig 3.6.
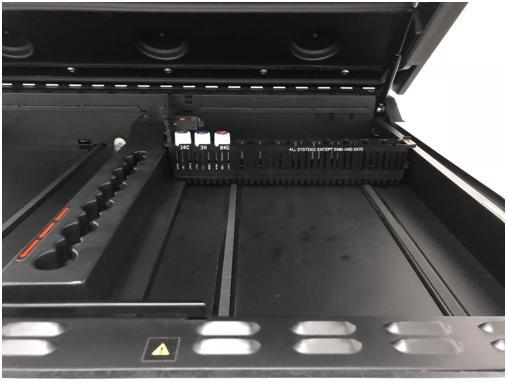
Dias et al. describe a method of using the liquid scintillation analyzer. They prepared a cocktail with100 ml of samples and evaporated without boiling it until dryness was achieved, and the substrate was dissolved in 10 ml of 0.1M nitric acid to produce Ultima Gold AB [34]. The solution was transferred to a vial and agitated for 3 minutes. Counting was carried out for 0-2000 channels and pulse shape determination was set at 128. Alpha efficiency was determined with a 237Np standard and beta efficiency was determined with 90Sr/ 90Y. Packard TRICARB 2700 LSC System was used to determine the background count. Standard equations were used to calculate the alpha and beta efficiency.
- Alpha Efficiency = (Alpha Standard Counting on Alpha Channel – Alpha Channel Background)/ Alpha Standard Activity x 60
- Alpha Activity = (Sample Counting on Alpha Channel – Alpha Channel Background)/ 60 x Alpha Efficiency x Sample Volume
- Beta Efficiency = [(Beta Standard Counting on Beta Channel – Beta Channel Background)/ Beta Standard Activity x 60] / 2
- Beta Activity = (Sample Counting on Beta Channel – Beta Channel Background)/ (60 x Beta Efficiency x Sample Volume)
The main objective of preparing aqueous solution samples is to mix a homogeneous solution that allows efficient energy transfer. Aqueous solutions are simple and provide the required solvent media for radioisotopes. Methods used to prepare aqueous samples are dissolution, extraction, and dissolution. In this research, the distillation method is used. Types of aqueous solutions are buffers, acids, alkalis, and others [36]. This research uses the type of acid of aqueous solution since nitric acid is used as an extractant, pH modifier, and solubilizer. In this research, the Ultima Gold AB cocktail was produced by adding 0.1M nitric acid.
List of References
M. James. Physics for radiation protection: a handbook. Wiley Publications (2008).
L. Hans, G. Kellerer. Radionuclides. Ullmann’s Encyclopedia of Industrial Chemistry (2011).
United Nations Scientific Committee on the Effects of Atomic Radiation. Web.
S. Sharma, B. Singh, V. Manchanda. Phytoremediation: Role of terrestrial plants and aquatic macrophytes in the remediation of radionuclides and heavy metal contaminated soil and water. Environmental Science and Pollution Research 22 (2015) 946-962.
AC. Patra, CG. Sumesh, S. Mohapatra, SK. Sahoo, RM. Tripathi, VD. Puranik. Long-term leaching of uranium from different waste matrices. Journal of Environmental Management 92 (2011) 919-925.
S. Jason, M. Kruk. Determination of 137Cs, 90Sr, 40K radionuclides in food grain and commercial food grain products. Journal of Radioanalytical and Nuclear Chemistry 289 (2011) 185-190.
A. Murad, XD. Zhou, P. Yi, D. Alshamsi, A. Aldahan, XL. Hou, ZB, Yu. Natural radioactivity in groundwater from the South-Eastern Arabian Peninsula and environmental implications. Environmental Monitoring and Assessment 186 (2014) 6157-6167 M. Caline. Web.
AM. Al-Amir, IF. Al-Hamarneh, T. Al-Abed, M. Awadallah. Natural radioactivity in tap water and associated age dependent dose and lifetime risk assessment in Amman, Jordan. Applied Radiation and Isotopes 70 (2012) 692–698.
DM. Al Shamsi. Natural radioactivity in groundwater, rocks and sediments from some areas in the UAE: Distribution, Sources and Environmental Impact. Dissertation United Arab Emirates University, 2014.
JC. Igwe, IC, Nnororm, M. Gbaruko. Kinetics of radionuclides and heavy metals behavior in soils: implications for plant growth. African Journal of Biotechnology 4 (2005) 1541-1547.
A. Baeza, J. Paniagua, M. Rufo, J. Guillén, A. Sterling. Seasonal variations in radionuclide transfer in a Mediterranean grazing-land ecosystem. Journal of Environment Radioactivity 55 (2001) 283–302.
MS. Al-Masri, B. Al-Akel, A. Nashawani, Y. Amin, KH. Khalifa, FA. Al-Ain. Transfer of 40K, 238U, 210Pb, and 210Po from soil to plant in various locations in south of Syria. Journal of Environmental Radioactivity, 99 (2008) 322-331.
NQ. Huy, TV. Luyen. Study on external exposure doses from terrestrial radioactivity in southern Vietnam. Radiation Protection Dosimetry Journal 118 (2005) 331-336.
S. Rihs, J. Prunier, B. Thien, D. Lemarchand, MC. Pierret, F. Chabaux. Using short-lived nuclides of the U and Th-series to probe the kinetics of colloid migration in forested soils. Geochimicaet Cosmochimica Acta 75 (2011) 7707-7724.
N. Chkir, A. Guendouz, K. Zouari, FH. Ammar, AS. Moulla. Uranium isotopes in groundwater from the continental intercalaire aquifer in Algerian Tunisian Sahara (Northern Africa). Journal of Environmental Radioactivity 100 (2009) 649-656.
B. Yarar, RB. Richter. The treatment and disposal of natural radioactive waste from the oil and natural gas industry – potentials and limitations. Mineral Processing 53 (2012) 58-71.
AQ. Ababneh, H. Al-Omari, M. Rasheed, T. Al-Najjar, AM. Ababneh. Assessment of gamma-emitting radionuclides in sediment cores from the Gulf of Aqaba, Red Sea. Radiation Protection Dosimetry 141 (2010) 289-298.
R. Rahman, A. Solodov. Analysis of natural and anthropogenic radionuclide content in palm date fruit of the United Arab Emirates: A Baseline Study. Health Physics 111 (2016) 465-470.
B. Fay. The environmental impact of the Gulf War. The Ecologist 21 (1991) 166-172.
KN. Jallad. Radioactive investigation of sand from the northern region of Kuwait. Environment and Natural Resources Research 3 (2013) 68-77.
H. Koch-Steindl, G. Pröhl. Considerations on the behavior of long-lived radionuclides in the soil. Radiation and Environmental Biophysics 40 (2001) 93-104.
AS. Okedeyi, AM. Gbadebo, AO. Mustapha. Effects of physical and chemical properties on natural radionuclides level in soil of quarry sites in Ogun State, Nigeria. Journal of Applied Sciences 14 (2014) 691-696.
C. Gil-García, A. Rigol, M. Vidal. New best estimates for radionuclide solid–liquid distribution coefficients in soils, part 1: radiostrontium and radiocaesium. Journal of Environmental Radioactivity 100 (2009) 690–696.
P. Österholm, M. Aström. Quantification of current and future leaching of sulfur and metals from boreal acid sulfate soils. Western Finland. Soil Research 42 (2004) 547–551.
H. Vandenhove, C. Gil-García, A. Rigol, M. Vidal. New best estimates for radionuclide solid–liquid distribution coefficients in soils. part 2. naturally occurring radionuclides. Journal of Environmental Radioactivity 100 (2009) 697–703.
G. Sohlenius, P. Saetre, S. Nordén, S. Grolander, S. Sheppard. Inferences about radionuclide mobility in soils based on the solid/liquid partition coefficients and soil properties. Ambio 42 (2013) 414–424.
Al. Abdulla. An initial radiation baseline study of urban environments in UAE. Thesis Khalifa University, UAE, 2016.
JM. McArthur, P. Ravenscroft, S. Safiulla, MF. Thirlwall. Arsenic in groundwater: testing pollution mechanisms for sedimentary aquifers in Bangladesh. Water Resources Research 37 (2001) 109-117.
S. Stephen, T. Shah, AE. Pillay, E. Siores. A study of kinetics of metal leaching from polypropylene matrix using a hyphenated mass spectrometric technique. Canadian Journal of Pure & Applied Sciences 8 (2014) 2925-2931.
R. Thomas. Practical guide to ICP-MS: a tutorial for beginners, 3 ed. CRC Press (2013).
F. Vanhaecke, P. Degryse. Isotopic analysis: fundamentals and applications using ICP-MS. John Wiley & Sons (2012). Web.
FF. Dias, MHT. Taddei, EMBD. Pontedeiro, VF. Jacomino. Total alpha and beta determination by liquid scintillation counting in water samples from a Brazilian intercomparison exercise. Proceedings of International Nuclear Atlantic Conference (2009) Held 27 September – 2 October 2009 at Rio de Janeiro, Brazil, 1-8. Web.
JA. Sanchez-Cabeza, L. Pujol. A rapid method for the simultaneous determination of gross alpha and beta activities in water samples using a low background liquid scintillation counter. Health Physics 68 (1995) 674-682.