Introduction
Magnetic resonance imagery, commonly referred to as MRI within the medical community is a non-invasive procedure and instrument that is utilized to aid in diagnostic exercises. The MRI produces cross-sectional images of a patient’s body through the use of magnetization and electromagnetic fields to produce a high resolution, and three-dimensional image of a patient’s body or tissue (Gomillion, et al. 2007).
The purpose of this article is to detail the theory behind the functionality of the MRI, its standard operation, the various advantages and disadvantages associated with its usage, as well as the basic construction of a typical MRI machine (Noll, 2001). This article will be divided into subsections, each of which is detailed in the table of contents. It is the aim of this article to provide its reader with a more accurate and complete summary of the medical use of magnetic resonance imagery.
Theoretical Basis of Magnetic Resonance Imagery
The human body, like all matter, is made up of a collection of atoms, which contain different numbers of subatomic particles – namely protons, neutrons, and electrons. Each element within the periodic table, as well as its associated isotopes, is created through various combinations of subatomic particles. These atoms or atomic particles are then arranged in a specific pattern to create simple to complex materials, including everything from a simple water molecule to complex nerve and brain tissue. It is these patterns, both atomic and subatomic, and their characteristics that allow the theory behind magnetic resonance imagery to operate (Noll, Gomillion, et. Al., 2001, 2007).
When a particular atomic particle has an uneven number of protons or neutrons, the electrical charge becomes unneutral. This neutrality leads to a slight magnetic field for each atom, as well as a minuscule electrical charge, much like a current of electrons that is passing through a wire loop. The magnetic field for each atomic particle can then be affected, and the resultant changes to the atom can then be measured. This is what happens, on a collective scale, when using the MRI machine (Huda, et Al, 2003).
Generally speaking, when these magnetized atomic particles are in a natural state, or outside a magnetic field, their magnetic orientation is highly randomized. However, once they are placed in a static magnetic field, the magnetic orientation of each atomic particle will orient themselves to be aligned with the prevailing direction of the charged particles within the static field. This initial alignment is crucial for the production of images since it is measuring the differences from this state of equilibrium that helps to determine the nature of the material being examined within the MRI device (Gomillion, et al, 2007).
In the operation of the MRI device, after the patient body (or other material being studied) is entered into the static magnetic field, and the randomized, and naturally magnetized atomic particles have had the opportunity to realign themselves, a secondary magnetic field is introduced into the equation, and at a perpendicular orientation to the subject and the static magnetic field (Huda, et Al, 2003). Upon the introduction of this second, perpendicular magnetic field, the atomic particles will experience something called excitation, or the increase of potential energy within their atomic mass, which in turn leads to slight alterations in their magnetic resonance and electrical fields. However, since atoms, like most matter, prefer to exist in low energy, more stable state, eventually the energy gained in the excited state will be released in a specific, two-stage decay process that is specific to each isotopic element. This process is referred to as the relaxation stage. The alteration between these two end stages will result in a slight, but measurable change to the tilt of magnetic orientation in relation to the static magnetic field and the introduced, perpendicular magnetic field (Noll, 2001). Please refer to Figure 1 for a graphical representation of a typical MRI machine in use.
The accurate measure of this change, as well as the time of decay, allow for the resonant frequency of a unique material to be determined through the use of the following equation:
f = -gB0
whereas f is equal to the resonant frequency of the atomic particles, “g” is equal to the gyromagnetic ratio, a constant specific to each particular isotope, and “B0” is equal to the magnitude of the applied magnetic field, given in Teslas. In general practice, most MRI machines are operated at a magnetic magnitude of somewhere between 0.5 and 3.0T (Noll, 2001, Huda, et al, 2003). Once this resonant frequency is calculated, and the time it takes for the atoms to return back to their unexcited state, the computer can create a cross-sectional image of the material being examined (in this case a patient) based upon the specific densities, and atomic composition of the material.
Since each isotopic element has a specific decay pattern, if all available isotopes were examined, the resultant image would be hopelessly complicated, and the computer would not be able to resolve the image. In order to eliminate this variable, the use of magnetic resonance imagery focus on one isotope. In most cases, the isotope chosen is that of the hydrogen ion or 1H. This is because the hydrogen ion is one of the most plentiful in the human body, and the fluctuations in magnetization experienced by the proton in the hydrogen ion are one of the easiest to measure. However, other isotopes for other elements have been used as well, including 13C, 23Na, and 19F (Gomillion, et al, 2007).
By measuring the difference, and directionality of the excited magnetized hydrogen atoms in relation to the relaxed states, and their approximate locations in respect to the two magnetic fields (the static and introduced), the computer can then generate a cross-sectional image of the material based on the relative differences in density presented. These cross-sectional images are then compiled through a layering process to create a three-dimensional image of the patient being examined (Gomillion, et Al, 2007). Since normal tissue, bone, and pathological tissue often have different densities, a trained medical professional can utilize this machine and technique to aid in the localization of such things as tumors, foreign bodies, and abnormal growth patterns.
Common Advantages and Disadvantages of the MRI
While the MRI is certainly a useful tool in the diagnosis of injury or disease it should be cautioned that it does have both advantages and disadvantages. The most common advantage cited is that of its non-evasive nature(Noll, 2001). However, it can be costly to operate, is often a very bulky machine, and often requires the patient to remain immobile for twenty minutes or more, a task that can be difficult depending on the mental and physical state of the patient being examined. There are also a number of safety precautions that must be understood and followed prior to, and during its operation.
First is the understanding the ferromagnetic materials will be affected by the strong magnetic fields utilized by the MRI. Because of this, objects such as keys, pens, needles, vials, paper clips, and other metallic objects have the potential to become projectiles due to the strong attractive and repelling magnetic forces. Therefore it is important to remove any and all objects that could be affected by the patient or any person working in the vicinity of the machine during operation (Noll, 2001).
Also, prior to usage, it must be determined if the patient has any implanted biological devices, especially if they are electronic in nature. The strong magnetic fields of the MRI have been known to significantly impede the operation of said devices, such as a pacemaker, and therefore an MRI scan is usually not recommended for such patients (Huda, et Al, 2003, Noll, 2001).
Conclusions
If utilized correctly, an MRI or magnetic resonance imaging machine can provide detailed, and informative three-dimensional images that can aid a medical professional in reaching the correct diagnosis prior to treatment (Gomillion, et al, 2007). While it does have a number of disadvantages, the ability to create an accurate and detailed three dimensional image through the use of magnetized atomic particles does indicate that its usefulness outweighs the costs in a number of cases.
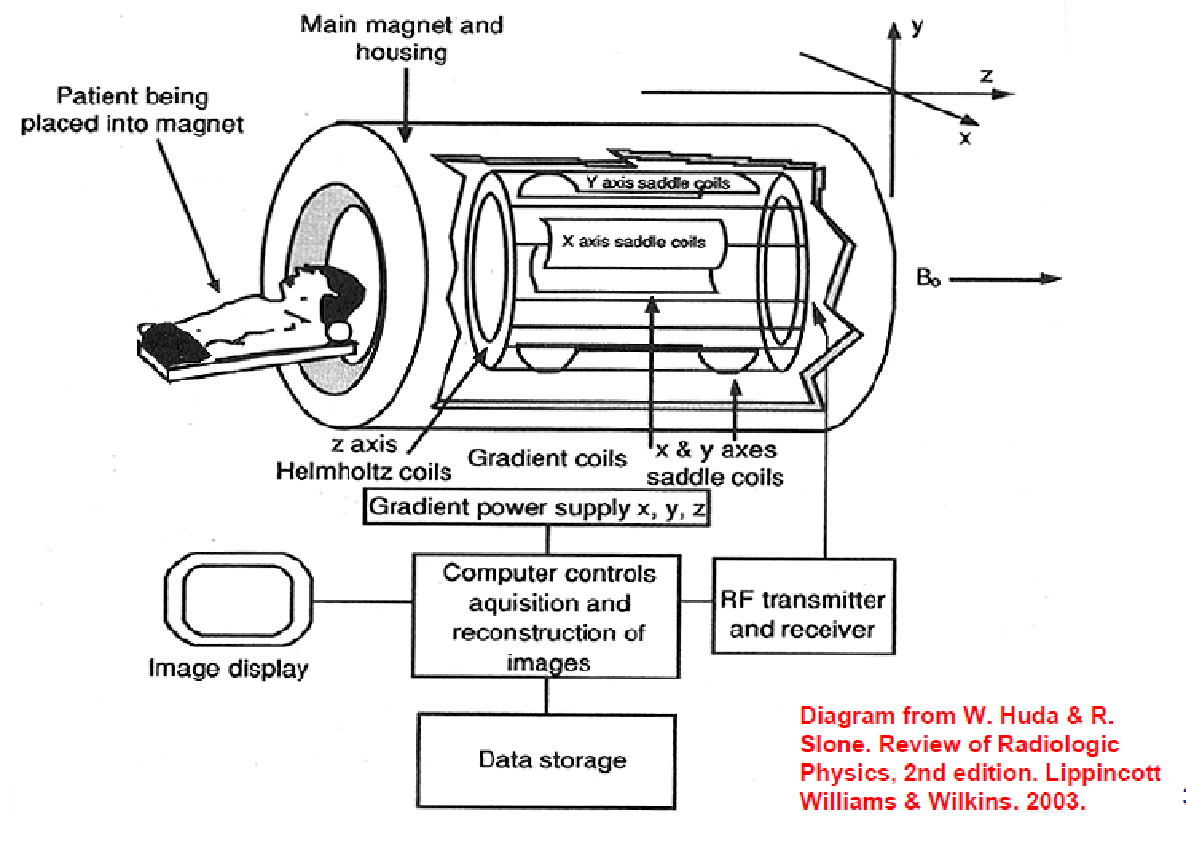
References
Huda, Walter, Slone, Richard Review of Radiologic Physics 2nd Edition Lippincott, Williams and Wilkins, Publishers 2003
Noll, Douglas, “A Primer on MRI and Functional MRI” Departments of Biomedical Engineering and Radiology, University of Michigan, Ann Arbor, MI 48109-2125 pp. 1-14 2001.
Gomillion, Matthew, Jung Hee Han “Chapter 61: Magnetic Resonance Imaging” Yao & Artusio’s Anaesthesiology: Problem-Oriented Patient Management Sixth Edition Fun-Sun Yao, Vinod, Malhotra, Manuel, Fontes, (eds.) Lippincott, Williams and Wilkins, Publishers 2007 pp. 1232-1248