Academic Honesty Statement
I declare that this is my work and has not been submitted in part or whole to any other learning institution.
Abstract
Water is a limited and threatened resource that is required for the sustenance of plant and animal life. A worldwide water crisis exists as shown by roughly 2 billion people who do not have access to clean drinking water. It is projected that a substantial fraction of the global population will face an acute water shortage in the coming decades. Additionally, the population and economic growth observed in recent years, coupled with lifestyle modifications and high living standards have increased the global water use threefold. Household activities lead to the generation of large quantities of wastewater.
Greywater is wastewater produced from household and office uses that do not cause fecal pollution. Examples of household activities that generate greywater include bathing, hand washing, and washing of clothes by hand or machines. As a result, greywater is relatively safe for reuse because it contains fewer pathogens than black water. In the face of the current water shortage, there is a need to safeguard clean water resources by reusing water.
The purpose of this project is to develop a greywater recycling system for landscape irrigation in hospitals and hotels. The system will be made up of a filtration component, a reverse osmosis system, and an ultraviolet disinfection unit. The entire project is expected to run for 20 weeks. It is expected that the successful installation of the proposed greywater treatment plant will produce high-quality greywater that meets British standards for recycled greywater use. It is hoped that using recycled greywater for landscape irrigation will improve the overall health of plants in the selected hospitals and hotels. Most importantly, it is anticipated that reusing recycled greywater for landscape irrigation will reduce the clean water demands for these institutions and avail clean water for potable uses.
Introduction
Greywater refers to wastewater generated by everyday uses that do lead to contamination with fecal waste. On the other hand, water generated from toilet use is regarded as âblack water.â Everyday activities that produce greywater include taking a bath, washing hands, and doing laundry. As a result, it is safer to reuse greywater than black water because greywater contains few disease-causing microorganisms. Greywater can be treated and used for agricultural purposes, flushing of toilets, landscaping, and other non-potable uses. Other terms used to refer to this type of water include sullage, gray water, greywater, and greywater.
The term greywater will be used throughout this project. Greywater contains low levels of pathogens and organic matter, which implies that it can be recycled without extensive treatment processes. Greywater composes more than half of the total water used in a home setting. However, it contains approximately 33% of the total organic matter and nutrients that are typically present in other forms of wastewater (Fountoulakis et al. 7070). These properties make greywater a good source for water recycling.
Water is a constrained resource that plays a vital role in the wellbeing of plants and animals because of its role in biological reactions. There is a universal water predicament as indicated by approximately 2 billion people who cannot find clean drinking water (Green et al. 108). It is projected that a substantial fraction of the global population will face an acute water shortage in the coming decades. Recent statistics show that the world population has increased significantly. Technological advancements have also led to economic growth and elevated living standards, which have tripled the global demand for water (Green et al. 108).
The water scarcity problem is further worsened by poor water quality attributed to contamination. Climate changes have also led to frequent droughts and a decrease in rainfall levels, which has aggravated the situation. The reuse of greywater in metropolitan water systems has a beneficial impact on the water provision system by lowering the requirement for clean water. Greywater reuse also lowers the pressure on wastewater systems by reducing the quantity of wastewater that needs to be treated.
This proposal describes a proposed greywater recycling system for irrigation and landscaping applications. The entire work consists of five chapters the first of which provides a background of the problem, a description of the project, and project objectives. Chapter 2 is a review of the literature concerning the problem of greywater, trends in water reuse, and methods used in the recycling of greywater globally as well as in the United Arab Emirates (UAE). Chapter 3 describes the proposed design, fabrication, simulation, and development of specs for the water recycling system. On the other hand, Chapters 4 and 5 provide the project management and quality assurance plans for the proposed greywater recycling system.
Background
Water recycling can be described as re-claiming treated wastewater for valuable intents, for example, irrigation of crops in agriculture, maintenance of landscapes, industrial processes, flushing of toilets, and refilling of groundwater basins (Green et al. 117). However, the treatment of wastewater for reuse should match the water quality needs of the intended processes. Recycling water for non-potable uses needs fewer treatment processes than salvaged water for drinking purposes.
Examples of non-potable uses of recycled water include the irrigation of landscape, agricultural farms, open parks, and golf courses; preparation of concrete for construction activities; and creation of man-made lakes. Industrial machines such as power plants and oil refineries can be cooled using recycled water. Domestic processes such as flushing of toilets and dust control can also make use of recycled water. Reusing water saves vast resources and finances. Also, recycling water according to the stipulated standards and regulations does not lead to human health complications. Water reclamation continues to be embraced because it is reported to be effective in creating a dependable water supply without posing a threat to public health.
The earth is a good example of a water recycling system. The earth is responsible for reclaiming water over millions of years through the natural water cycle (Leong et al. 3014). However, the term water recycling often denotes undertakings that take advantage of technology to hasten the natural processes involved in water reclamation. Two types of water recycling occur the first of which is unplanned water reclamation. A typical example of this form of recycling is when towns obtain their water supplies from waterways that receive wastewater effluents from those towns. In such a scenario, water from these waterways is reused, purified, and channeled back into the water supply several times before it can be used by the final downstream user. Planned projects, conversely, are systems designed to reclaim a recycled water supply constructively.
Greywater may be recycled and reused on the site where it is produced. For instance, an industrial plant that reuses water used to cool its machines. Greywater is mainly reused on-site, especially for the irrigation of landscape. However, there is a need to use innocuous and low-sodium detergents and personal care items to safeguard the vegetation when reusing greywater for irrigation (Leong et al. 3015).
As a result, the National Science Foundation (NSF) International has set up a wastewater treatment commission to look into on-site suburban and industrial greywater treatment systems. One requirement is that households should have different wastewater lines for black water and greywater. Meeting this requirement is possible in new constructions. However, setting up such systems in pre-existing buildings can be an uphill task.
Figure 1 is a treatment and application diagram that indicates different water treatment processes and the recommended uses at each treatment stage. Processes with a likelihood of human exposure to recycled water require additional treatments. Poor treatment of water is linked to disease-causing pathogens and other pollutants that can cause adverse health outcomes.
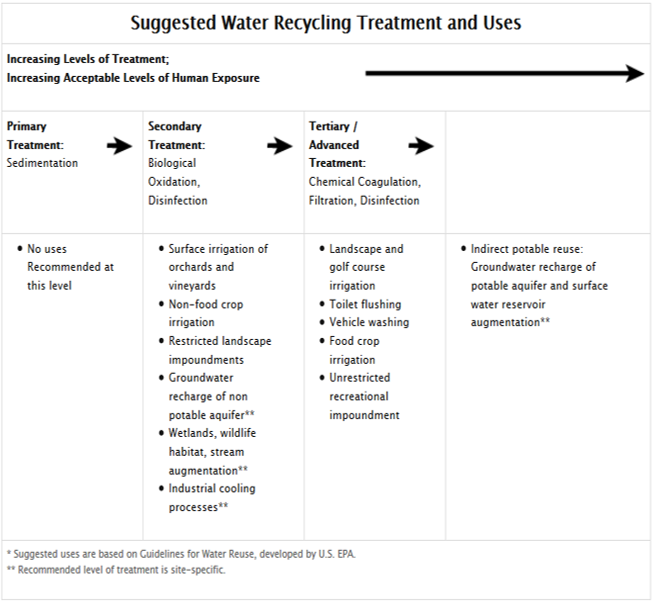
Although most water recycling projects have been developed to meet non-potable water demands, several projects use recycled water indirectly for potable purposes. These projects include recharging groundwater aquifers and augmenting surface water reservoirs with recycled water. In groundwater recharge projects, recycled water can be spread or injected into groundwater aquifers to augment groundwater supplies, and to prevent saltwater intrusion in coastal areas. Progress in the wastewater treatment expertise and health research of indirect potable reuse is likely to lead to planned indirect potable reuse of wastewater.
Using greywater for irrigation and flushing of toilets at dispersed sites lowers the amount of fit-to-drink water that is dispensed to these sites. Greywater for irrigation reduces the amount of fertilizer required to ensure the nourishment of garden plants. Another benefit is a reduction in quantities of wastewater that is produced, transported, and purified at wastewater treatment centers. Consequently, water recycling saves water, energy, and financial resources.
Widespread use of decentralized water recycling systems is in the arid areas that are characterized by longstanding drought conditions. Effective greywater systems are capable of meeting half the water needs in an estate by providing water for landscaping. Reclamation of greywater saves clean, drinkable water and decreases the amount of wastewater in septic tanks and wastewater treatment systems.
Apart from providing a reliable water supply, the reclamation of greywater has vast environmental advantages. Water reuse offers an additional water source, thus preventing the redirecting of water from delicate ecosystems. It also lowers wastewater effluents and minimizes pollution. Reclaimed water is a valuable resource in the creation and enhancement of wetlands and habitations on riverbanks (riparian habitats). Adequate water flow to various habitats is vital to the survival and reproduction of plants, animals, and fish. Wetlands serve numerous purposes, including being habitats for wildlife, enhancing water quality, and providing breeding grounds for fisheries. Water flow in streams can be improved using reclaimed water to sustain aquatic habitats.
The redirecting of water from natural ecosystems for domestic, industrial, and agricultural use interferes with the flow of water, which depreciates the quality of water and the wellbeing of ecosystems. Recycled water helps to meet domestic water use demands, which frees substantial quantities of water to vital ecosystems.
Reusing water minimizes the emission of effluents to water bodies that could be easily upset by disturbances in water quality. In some instances, the push for water recycling stems from a need to reduce wastewater emission to water bodies such as estuaries, streams, or oceans. For example, wastewater recycling prevents the transformation of salt-water marsh into the brackish marsh, which safeguards the habitat of endangered species such as the California clapper rail, Belding’s Savannah sparrow, and salt marsh harvest mouse.
Water reclamation minimizes pollution. Substances that can be contaminants when released into a body of water can be reused constructively for irrigation. Greywater is a rich source of nutrients such as nitrogen. Making use of greywater for irrigation of landscapes and agricultural lands provides extra nutrients to the plants, thus eliminating the need for the application of synthetic fertilizers. This use also prevents the discharge of nitrogen-rich water-to-water bodies, which minimizes the number of pollutants in these waterways.
Recycling water also saves energy. To meet water demands, water needs to be extracted, purified and conveyed over long distances to the point of use. These processes consume a lot of energy. The extraction of water from groundwater sources further lowers the level of the water as more water is extracted. As a result, extra energy is needed to pump the water to the surface. On-site reclamation of water eliminates the need for energy use to convey water over long distances or to pump water from an underground reservoir. Matching the quality of water to specific needs also lowers the energy required to purify the water.
For instance, the water quality needs for drinking water are more stringent than the water quality required for flushing toilets, which requires minimal energy to achieve. Also, the recycling of greywater consumes less energy compared to water purification using desalination systems.
The growing water demand enhances the importance of water recycling in the overall water supply. As much as water recycling is a beneficial and justifiable approach to wastewater management, in the long run, the purification of wastewater at centralized plants is more costly than the reuse of greywater. The high costs are attributed to the fitting of distribution systems. Public misconceptions, regulatory standards, and institutional barriers may also get in the way of water recycling efforts. Therefore, it is necessary to conduct adequate groundwork to establish measures to circumvent these barriers. A useful strategy is ensuring public awareness in the early planning stages of water recycling projects and involving the public in the planning process.
Description
This project aims at designing a system of greywater recycling for hospitals and hotels. The hospitality industry has numerous water uses that lead to greywater generation, including food preparation and clean up, cleaning of rooms, showering, and laundry. Also, the landscape of luxurious hotels contributes to their beauty, which plays an important role in attracting clientele. The uses of water in hospital settings are also similar to those in the hospitality industry. Therefore, a significant amount of water is needed to sustain these processes and ensure effective service delivery. However, these industries (hospitality and healthcare) also involve other uses of water that lead to the production of black water. The situation is further aggravated in healthcare settings due to the emission of pathogenic microbes alongside black water.
Therefore, there is a need to consider strategies of separating greywater and black water in these settings to develop an effective greywater recycling system that will enable the safe use of greywater for irrigation, which is the focus of this project.
Goal/Objectives
The overall purpose of this project proposal is to design a system aimed at treating greywater for irrigation and landscaping applications. Specific objectives to attain the overall goal will include:
- Designing a greywater recycling system with reverse osmosis using British Standards.
- Describing the management of the project.
- Developing quality control measures to guide the operations of the system.
Literature Review
Water Scarcity
Clean, freshwater is a vital requirement for animal, plant, and human life. Water consists of approximately 70% of planet earth. However, only 3% of the water is available for human consumption in the form of freshwater. To make matters worse, about 66% of the freshwater reserve is hidden in the form of ice and is unavailable for use. Additionally, water bodies are either drying up or becoming too polluted to provide quality water to meet the demands.
The term water scarcity denotes the disparity between the availability of water in the form of natural overflow and water needs over a definite time and region (Schewe et al., 3245). Water scarcity can be demand-driven or population-driven. Demand-driven water scarcity is stated as the actual water expended compared to the available water, whereas population-driven water scarcity is conveyed as the amount of water available per person annually.
Water scarcity hinders proper sanitation practices, which predisposes populations to water and food-borne infections such as cholera and typhoid. It is estimated that 2 million people succumb to these illnesses annually (Cassini et al., 22). Water scarcity also threatens food security due to incessant droughts and inadequate water to sustain agricultural efforts. Water scarcity alongside its related upshots is identified as an important global risk.
The population affected by water scarcity is reported to increase from 473 million to approximately 2.6 billion over 40 years (Veldkamp et al. 19). The current pressure on water resources has been worsened by population growth, socioeconomic conditions, and climate changes. Different studies have used population estimations and simulated climate changes to predict the impact of these factors on water scarcity, which is expected to worsen as time progresses (Gosling and Nigel 372).
If not tackled properly, the global water scarcity could debilitate efforts towards fighting world hunger. Consequently, there is a need to adapt to this shortage and modify our water usage patterns. Governments and organizations charged with handling water resources have been compelled to adapt to the water scarcity conditions. Some of the measures used to adapt to the existing water shortage include water rationing, generation of clean, freshwater through desalination and purification of water, and recycling of wastewater. Other measures include harvesting rainwater, promoting the sustainable use of groundwater by regionalizing water management, embracing of efficient water distribution machinery, and improving the efficiency of irrigation strategies.
Trends in Water Use
Economic growth and food production are dependent on the balanced use of global water resources. Nevertheless, there is an overuse of surface water and groundwater wherewithal globally, which is attributed to overreliance on non-renewable groundwater resources (Veldkamp et al. 19). This trend creates substantial uncertainty about the sustainability of local water supply and its related food production through irrigation. Agriculture is the pivot of the waterâfood relationship. As a result, irrigation is the leading consumer of freshwater globally, using up to 70% of all water. In developing countries, the agricultural sector consumes up to 90% of water.
Electrical energy is the predominant form of energy globally. Significant amounts of water are used in the generation of hydroelectric power. The use of water for the generation of electricity has increased fourfold in the last six centuries. The thermoelectric power industry also uses water to cool the equipment that generates electricity. However, most of this water is cooled and reused as opposed to irrigation where much of the water is used up by plants or lost to the environment by evaporation and transpiration.
In the arid regions of the world such as the Middle East, an uncontrolled demand for water for agricultural use has caused groundwater overâdrafting, which has led to significant reductions in water quality, land degradation, and salinization (Mlih et al. 77). The United Arab Emirates is among the countries that lead to water scarcity worldwide. Nevertheless, the country still records the world’s highest water consumption rates at 550 liters a day (Giwa and Abdallah 721). Additionally, the country is facing a tremendous population increase, which has further raised the water demand. The high water demand in the UAE is met by groundwater, desalinated water, and retreated water. Water use in the UAE is divided into three areas: private homes, agriculture, and industry.
Private households account for approximately a quarter of the entire water consumption in the UAE. The use of air conditioning systems is widespread because of the extreme temperatures experienced in the country all year round. Air conditioning systems use significant quantities of energy as well as water in the form of chilled water pumps (Giusti and Almoosawi 535). The country also reports a high per capita use of bottled water of 285 liters annually (Giwa and Abdallah 721).
Desalinated water is commonly used to fill the bottles at a cost of approximately 12 billion AED per annum. It is also reported that making one liter of bottled water uses up three liters of normal water. Water management endeavors in this country are thwarted by water misuse. For example, watering of gardens through spray irrigation is reported to expend between 12 and 15 liters of water per meter squared daily. Water conservation measures in private households include creating awareness of water-saving measures, instituting novel water tariff systems that use water meters, providing safe drinking water through taps, and the establishment of efficient methods of irrigation, for example, subsurface drip irrigation.
The agricultural sector contributes to 67% of water use in the UAE (Giwa and Abdallah 721). The population of upsurge has increased food demand, which has mounted extra pressure on the water resources. Low irrigation efficiency is responsible for water wastage in the agricultural sector. One-third of the water used for irrigation is lost to the environment through evaporation. Drip irrigation systems (Figure 2) that use less than half of the water used in overhead sprinklers (Figure 3) have been introduced by the government. Farming trends are also shifting away from water-intensive plants. Experiments are underway to look into the use of wastewater for irrigation.
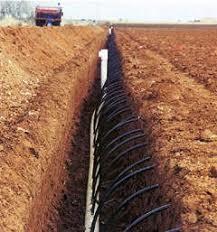
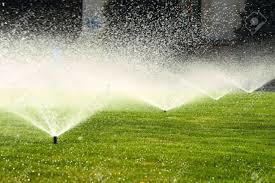
Industries use approximately 9% of the countryâs water consumption. The main uses of water include cooling and cleaning of machinery. This water is released into surrounding environments, leading to pollution. However, the resultant wastewater can also be used for irrigation due to government efforts to reuse wastewater. In the emirate of Abu Dhabi, approximately 600 million cubic meters of treated wastewater are generated every year (Giwa and Abdallah 722). However, only 352 million cubic meters are used for cooling and landscaping.
Greywater Exposure Risk to Humans and Ecosystems
Everyday uses of greywater include non-potable uses such as landscape irrigation, washing, and flushing of toilets. The reclamation of greywater for these purposes has beneficial impacts on the potable water supply because it alleviates the demand for clean water for tasks that can benefit from recycled water. However, the possible presence of different contaminants in greywater creates unease about its uses. Greywater use can lead to human contact with the water as well as unintentional ingestion exposures.
Groundwater recharge of greywater can also interfere with potable uses as well as have adverse environmental consequences for plants and soils in irrigated areas. The concerns of greywater reuse can be worsened by the lack of federal rules for its use and discrepancies in state and local guidelines for the beneficial use of greywater.
The chemical and microbial pollutants in greywater can bring about adverse health effects if one is exposed to them for prolonged periods. These effects could be acute or chronic. Chronic effects arise from recurrent, longstanding exposure to contaminated water, especially through ingestion. Various forms of cancer and reproductive complications are some of the chronic health effects of exposure to contaminated water. Acute infections, conversely, occur immediately following one or few contacts with contaminants.
Human pathogenic microorganisms are linked to acute infections such as stomach flu, skin and respiratory contagions, and inflammation of the conjunctiva. However, few studies are reporting the adverse health effects of greywater use. For example, Sharvelle et al. (3800) examined the health records of 15 states that permit the use of greywater in the United States. They noted that there were no illnesses attributed to greywater systems. These studies indicate that the risk posed by greywater use is unlikely to be big.
Nevertheless, waterborne infectious diseases tend to be underreported. Therefore, the use of recycled greywater should be directed by specific regulations, assessment of potential risk, and development of risk mitigation measures. When measuring the possible risk presented by greywater reuse, several factors need to be taken into consideration. It is worth noting that possible risks differ according to the quality of the water, the intended use, probable avenues of exposure to the water, and features of the surroundings where the water will be used. Moreover, one should know that it is impossible to eliminate all the risks related to the use of recycled greywater. Nevertheless, a comprehension of the dangers posed by greywater can inform the decision-making process, inform stakeholders, and direct the responsible use of greywater.
The initial stage in the risk assessment process is conducting a hazard estimation. This process entails identifying all contaminants of concern. The type and quantities of pollutants differ based on the sources of greywater and the end-use (Bichai and Ashbolt 454). Since greywater is obtained from household wastes, the contaminants of interest include pathogenic microbes found in humans and chemicals used in the manufacture of soaps, detergents, and cosmetics. Similarly, the quality of greywater varies from place to place depending on the lifestyles, health standing, and occupations of the people who live in those areas.
For example, greywater from a farmerâs home is likely to be contaminated by farm chemicals such as pesticides and herbicides. However, large-scale projects such as multi-residential establishments are likely to have constant water quality because discrepancies in impurity overloads are averages of many households (National Academies of Sciences, Engineering, and Medicine 64). Since greywater quality differs significantly, there is a need to indicate the expected contaminants in different situations.
Table 1 provides water quality measurements of concentrations of each component that can be expected in greywater from different sources. However, having contaminants in wastewater does not automatically mean that there is a significant risk of infection. The precise usage of the water is what determines the magnitude of risk. The risk of infection is considerably low when there is a minimal possibility of human exposure, for example, when using greywater in subsurface irrigation. Using greywater for spray irrigation increases the risk of ingestion of the contaminated water.
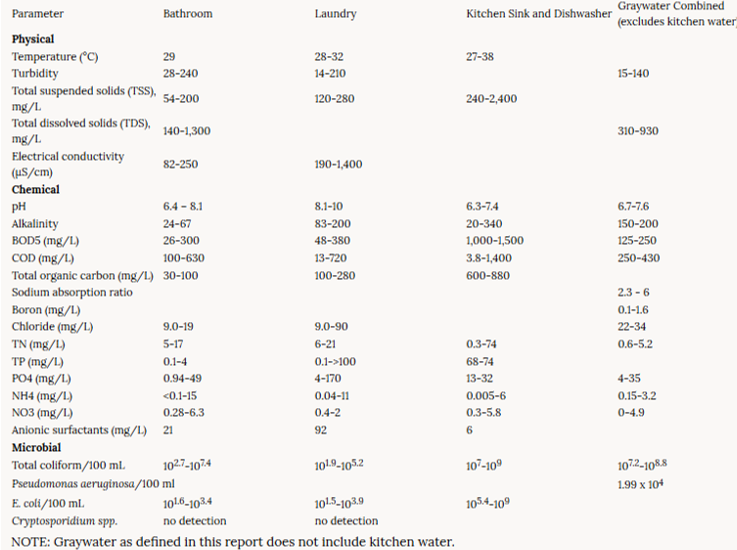
Table 1 also provides water quality information from kitchen sources. Kitchen greywater has high amounts of solids, organic substances, and indicator organisms. It is for these reasons that kitchen water sources are normally left out from greywater collection systems.
The human fecal material may contain a range of disease-causing pathogens. The availability of these pathogens in greywater is subject to the specific sources that contribute to the water. For instance, water from showers and plunge baths may be full of disease-causing microbes such as bacteria, helminths, protozoa, and viruses (National Academies of Sciences, Engineering, and Medicine 65). As a result, gastroenteritis is the most common health problem attributed to direct contact with greywater containing waterborne pathogens. Other potential health problems include encephalitis, hepatitis, and myocarditis.
Fecal pointer bacteria, for example, fecal coliform bacteria and total coliform bacteria are usually employed as indicators for the existence of pathogenic microbes in greywater. The concentrations of these bacteria are valuable in guiding the treatment needs for various end uses.
Nonetheless, the discovery of indicator bacteria does guarantee the presence of pathogenic organisms in wastewater. Since pathogens are only expelled by diseased persons, greywater is likely to have more pathogens when many people contribute to the greywater than when a small number of people contribute. Then again, even in waste watercourses to which a small number of people play a part, the concentration of pathogenic microbes may be very high when a disease-ridden individual is defecating pathogens because of the apparent lack of dilution. It is also worth noting that the absence of indicator microorganisms is also not a guarantee of the absence of pathogenic microbes in water.
This observation is connected to the higher persistence of pathogenic microorganisms compared to the indicator microorganisms. Bacteria such as Staphylococcus aureus and Pseudomonas aeruginosa, which are responsible for skin infections, may also be found in greywater (National Academies of Sciences, Engineering, and Medicine 64). Table 2 provides a summary of reported concentrations of microbial indicators and pathogens in greywater.
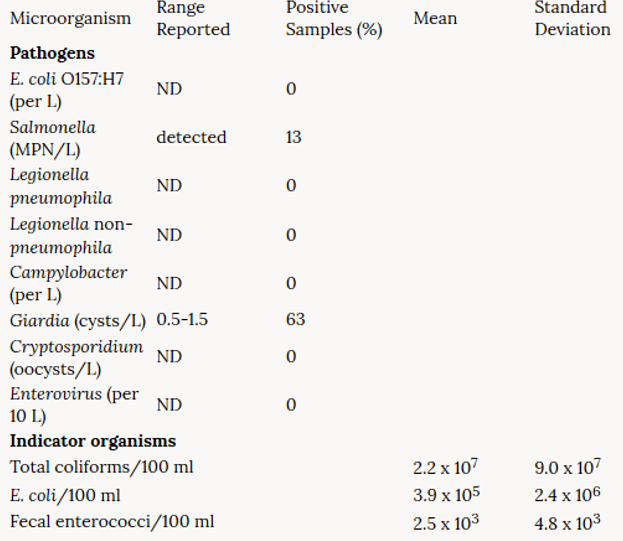
The quantity of organic matter in wastewater is measured using total organic carbon (TOC). Biochemical oxygen demand (BOD) of wastewater is an estimation of its degradability (National Academies of Sciences, Engineering, and Medicine 64). These factors can point towards the likelihood of oxygen exhaustion as a result of the microbial breakdown of organic substances in the course of transport and storage of greywater. Oxygen depletion may result in hypoxia and the production of sulfide. Additionally, elevated levels of biodegradable organic material in greywater limit the storage of greywater, its use for flushing of toilets, or in decorative fountains due to the prospect of microbial growth.
Xenobiotic organic chemicals are other contaminants that can be found in greywater. The most common examples are personal care products and everyday chemicals. However, there is limited information regarding the incidence of these chemicals in greywater sources. Small quantities of organic chemicals such as household chemicals, drug constituents, and chemicals that affect the endocrine system have been reported in domestic wastewater on a small scale.
However, greywater constituents differ from wastewater such that greywater contains mainly personal care products and antimicrobial agents used in the manufacture of hand soap as indicated in Table 3. These chemicals present possible worries for irrigation uses of greywater. Inorganic ions, for instance, sodium, chloride, and boron may have negative impacts on vegetation if present at high concentrations. Studies show that soils irrigated with greywater contain high amounts of sodium compared to soils watered using potable water. Laundry detergents and other cleaning agents contribute to boron identified in greywater.
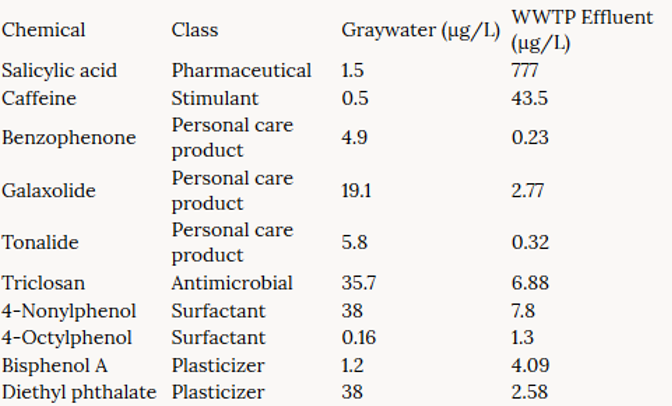
Risk Management Options for Greywater
The risk management measures for greywater use depend on the type of risk posed. One such risk is cross-connections. Currently, no greywater cross-connections have been reported. However, inadvertent or deliberate cross-connections between non-potable and clean water streams are a potential public health problem, especially for water supplies that have not undergone disinfection. Such an incident has been reported in the past in Melbourne when workers in a water company fell ill after taking water from a tap within the facility. An investigation revealed that a cross-connection had been made between potable water systems and non-potable recycled water (Murphy et al. 650). To manage this risk, all pipes conveying reclaimed water should be labeled appropriately.
There are concerns regarding the leaching of greywater components to groundwater when greywater is used in irrigation. This risk is increased when water is applied to large areas at rates that exceed the needed based on evapotranspiration. Components of interest include salts of sodium, chlorine, and boron, pathogens, nitrogen, and organic compounds from cleaning agents. Nevertheless, the actual human health risk depends on factors such as the concentrations of these compounds in greywater, the frequency of irrigation, the degradability of these compounds, and the sorption capacity of the contaminants.
Water source aspects such as soil and aquifer physiognomies that influence the movement of the contaminant, the depth of the water table, and the distance between the point of groundwater withdrawal and irrigated land also play a role in greywater contamination of water sources. The best way to manage this risk is by reducing the concentrations of contaminants in recycled greywater before using it for irrigation.
Greywater projects should also evaluate the possible toxicological effects of greywater on aquatic life, plants, and soil. High quantities of sodium cause salinity, which affects plant health and soil structure. High salt levels reduce the permeability of the soil and increase the susceptibility of soil to erosion. The effects of salinity are magnified in arid areas with high evapotranspiration rates and limited rainfall to wash away salt build-up from the soil. Therefore, it is necessary to ascertain the quality of treated greywater before releasing it for irrigation using the guidelines indicated in table 4.
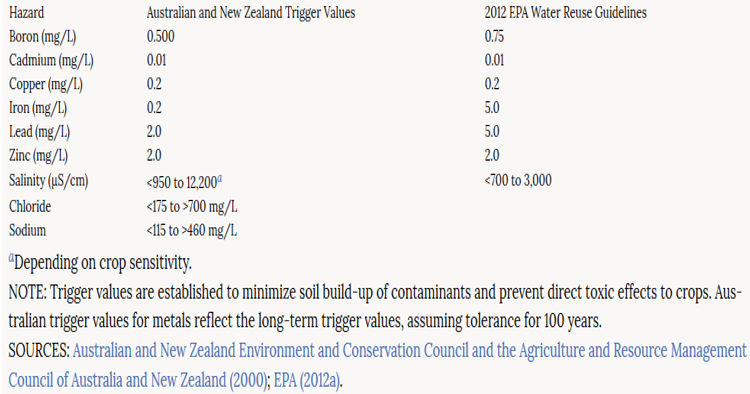
British Standards for the Treatment of Greywater
A wastewater treatment plant may be referred to as a system. Greywater systems in terms of intricacy and size, and can be classified into six categories based on the treatment modality used. The first group is direct reuse systems where wastewater does not undergo any treatment process. These systems consist of gadgets that collect greywater from the appliances and convey it to the usage points without treatment or storage. An example is a greywater diverter. Greywater may be used without treatment as long as prolonged storage is not needed because the quality of greywater depreciates at an alarming rate (Cook 231). However, untreated greywater usage is limited to sub-surface irrigation and non-spray uses.
The second group comprises short retention systems that use uncomplicated filtration or treatment methods, for example, skimming of fragments on the surface of greywater and letting large particles settle at the bottom of the tank. The treated water is also used within a short time of treatment to avoid the development of foul odors.
The third category is the basic physical or chemical greywater treatment systems. A filter is used to eliminate debris from the water before storage, whereas chemical agents such as chlorine or bromine are used to prevent the growth of bacteria during growth. The fourth class is biological systems where aerobic or anaerobic bacteria are used to digest any undesirable organic matter in greywater. Aquatic plants or manual pumps can be used to introduce air into the water in the case of aerobic treatment. The fifth category consists of biomechanical systems, which combine biological and physical means to purify water.
Organic matter may be removed by introducing microbial cultures, whereas solid particles may be left to sediment. The growth of microbial cultures is enhanced by passing oxygen through the collected water. These systems are the most advanced greywater treatment techniques. When any of the five treatment methods are combined during treatment, a hybrid system is formed.
Overall, greywater systems should be planned in such a manner that the treated water is fit for the intended purpose to avoid unnecessary health risks. Standard greywater treatment systems should include biological, filtration, and disinfection steps to ensure that the ultimate product is stable and that it conforms to the required quality standards. Disinfection is often done as the final step at the treated greywater storage and distribution tank to ensure that the water is sterile during the storage period.
For recycled greywater meant for flushing toilets, a blue dye is often added to the water. The purpose of the dye is to alert users that the water is not potable. Additionally, the coloring minimizes chances the risk of making cross-connections as well as misusing the greywater. However, the color should be made from a safe and environmentally friendly food-grade dye.
Greywater treatment systems have different capacities. However, different factors influence the type and treatment capacity of greywater recycling systems. Examples of such factors include the water quality requirements for the intended use, the demand for recycled greywater, and the anticipated yield of recycled water. The demand and yield of greywater are in turn determined by frequency and type of planned use, the volume of water used for each application, as well as the discharge figures for appliances connected for reuse. It is also important to consider the highest treatment rate and capability of the plant.
The storing of treated greywater needs to be restricted such that the water is produced for immediate use. If need be, treated greywater should be stored for a maximum of 24 hours. On the other hand, untreated greywater should be stored for less than 24 hours.
An efficient greywater recycling system should have distinct sanitary pipework that links the collection pieces of equipment such as showers and washbasins to the greywater recycling system by gravity. However, pumps can be considered in cases where pumps are not feasible, for example, in single-story buildings. The materials used should have a similar quality and structure as those used for sanitary plumbing systems. Caution should be taken to ensure that no black water, harmful chemicals, or polluted water enter the greywater recycling system. There should be room for connection of bypass pipes and valves to facilitate the flow of the collected greywater to the sewerage system during repairs, obstruction, or system isolation. Additionally, all pipes carrying greywater should be marked clearly.
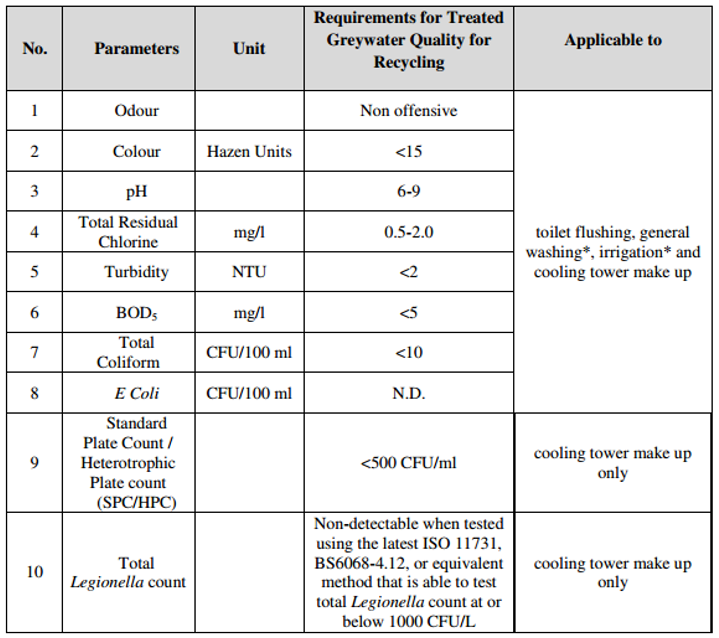
A useful aspect when recycling greywater is to treat the water to an extent that matches the target use of the treated water (see table 5) to evade unwarranted treatment costs. Often, the treatment of greywater combines biological, physical, and chemical procedures. Biological action makes use of microbes suspended in the wastewater or immobilized onto a support medium to aid the removal of organic matter from the wastewater. On the other hand, physical treatment gets rid of waste by percolation through granulated or solid media, for example, membrane filtration. In chemical treatment, certain chemicals are added to the wastewater to precipitate specific substances or adsorbing them onto a medium. Details regarding each of these treatments are provided as follows.
Microfiltration, Ultrafiltration, and Nanofiltration
Microfiltration is employed in the clarification of solids or the elimination of bacteria, protozoa, and tiny floating solids. Most membrane filtration processes use microfiltration alone or as an initial step before reverse osmosis. The pores of a microfiltration membrane have a diameter of 0.1 microns, which traps and concentrates particles in this size range. Using microfiltration alongside post-filtration and ultraviolet (UV) disinfection is highly effective in the elimination of viruses because it gets rid of particles that protect viruses from UV energy. Ultrafiltration and nanofiltration are beneficial in lowering the BOD of wastewater, detaching oil from used water, and reducing pathogens.
These methods also get rid of natural and man-made organic compounds, inorganic substances, and by-products of decontamination. The pore sizes in ultrafiltration membranes are 0.01 microns in diameter, whereas nanofiltration membranes have pore sizes of 0.001 microns (Werber et al. 16018). Macromolecules such as sugars and proteins fall within this size range and are entrapped in nanofiltration membranes.
Membrane Bioreactors (MBR)
Membrane bioreactors merge standard biological treatment processes with membrane separation to generate treated greywater of exceptional quality. Other advantages of MBR systems include size and low operational requirements. MBR systems are compact hence save space. Their operational costs are also lower than other conventional treatment systems. MBR systems are reported to generate treated greywater that meets all existing guidelines for greywater for toilet flushing.
Biologically Activated Carbon (BAC) Filtration
Activated carbon filters work by adsorbing organic chemicals in wastewater. As a result, such systems are effective in eliminating pollutants such as drug compounds, pesticides, by-products of disinfection, toxic cyanobacterial compounds, and herbicides. The adsorption efficiency of activated carbon filters can be augmented by pre-treating wastewater via ozonation. Ozonation disintegrates large organic compounds into smaller particles that are easily absorbed. The activated carbon filters can be regenerated by burning the contaminants.
Dissolved Air Flotation
In this method, a chemical flocculant is added to wastewater to form floc particles. Water purification by dissolved air flotation entails introducing air into water, which leads to the formation of very small bubbles that stick to floc particles. The floc particles become buoyant and float to the surface of the water to be skimmed off.
Disinfection
Disinfection is a crucial treatment element of greywater recycling systems because it ensures that the treated greywater is suitable for use. Disinfection safeguards human health and the environment. Moreover, disinfection is mandatory to meet the water quality standards for the use of greywater for irrigation. Disinfection alternatives for greywater include chlorination, UV radiation, and sophisticated oxidation processes entailing the use of ozone or hydrogen peroxide.
Each disinfection method has its advantages and disadvantages in various circumstances. Sometimes it may be necessary to combine several methods to yield the best outcome. The British standards of greywater disinfection require all disinfection systems to be automated with alarm bells and spontaneous shut-off of the remedied greywater supply in the event of failure. Additionally, all chemicals used in the disinfection process should be used and stored according to the relevant regulations and guidelines. For example, chemicals containing chlorine should be kept separately ensuring adequate distance with acidic reagents to avoid accidental mixing.
Chlorination
Chlorination involves the use of chlorine-containing chemicals such as sodium hypochlorite or chlorine dioxide. Dosage and contact time are critical components that determine the efficacy of chlorination as a method of disinfecting greywater. These components are determined by the target use of treated greywater and the physical characteristics of the greywater, for instance, pH and BOD. The extent of the contamination by organic substances, manganese, iron, and ammonia determines the amount of chlorine required to disinfect the water, which is referred to as the chlorine demand.
Chlorine reacts with ammonia in wastewater to form chloramines, which complicate the determination of free versus bound chlorine. The main shortcoming of chlorination is that it is ineffective at high pH levels. Therefore, it is important to consider these aspects when creating a treatment program for greywater recycling to ensure adequate disinfection of treated wastewater. Another shortcoming of chlorination is its relative ineffectiveness at incapacitating certain pathogens, for example, the oocysts of Cryptosporidium. Chlorine may also persist in treated greywater. The leftover chlorine may be lethal to sensitive organisms that are exposed to chlorinated greywater.
Ultraviolet Irradiation
Ultraviolet radiations disinfect greywater by triggering a photochemical reaction that destroys DNA molecules within microbes. The energy absorbed generates photoproducts, for instance, thymine dimers on the nucleic acid strand. These dimers hinder the replication of DNA, thereby leading to the inactivation of microorganisms. Consequently, microbial cells cannot divide and multiply. The extent of cell damage is directly proportional to the amount of UV energy taken in by the cell as well as the cell’s endurance of UV energy.
The effective UV dosage is a result of the strength of UV rays and the length of exposure and is stated as milliwatts seconds per square centimeter (mW.s/cm2). The main advantage of UV disinfection is that it does not generate noxious by-products. However, this method is only suitable for greywater containing low quantities of suspended solids, low turbidity, and minimal color. It may also be necessary to add a chemical compound such as chlorine following UV disinfection to prevent the re-growth of bacteria in the distribution system.
Oxidation Processes
Oxidants used in the disinfection of greywater include ozone, hydrogen peroxide, or chlorine dioxide. Of the mentioned oxidation processes, ozonation is the most common. For indoor premises, the level of ozone should not exceed the guideline (Albalawneh and Tsun-Kuo 18). A main limitation of ozonation is that it generates appreciable amounts of disinfection by-products that may have deleterious health consequences when inhaled or ingested. Additionally, ozone gas is highly toxic. Therefore, ozone-generating facilities require strict management practices as stipulated by occupational health and safety provisions. If ozonated greywater is expected to stay for long before being used, chlorination may be needed to inhibit microbial re-growth.
Greywater Treatment Methods in the UAE
UAE, freshwater is a valuable resource. Considerable economic and population growth in the UAE has resulted in an extraordinary increase in water consumption notwithstanding the low natural accessibility to water in the country. However, significant amounts of freshwater are flushed down the drainpipe each day. It is estimated that approximately 30% of clean water is used in flushing toilets. Such usage of water is inexcusable in the face of diminishing groundwater supplies. The UAE relies heavily on the generation of freshwater through desalination techniques to meet its water demands, which has reduced dependence on groundwater sources.
Nevertheless, efforts to minimize water consumption in the country are still needed not only to economize resources but also to lower pressure on desalination plants and minimize the accompanying environmental effects. A proposed solution is using recycled greywater to flush toilets in residential buildings. The proposed solution suggests that wastewater from showers and washing machines should be purified in a system within the building and used to flush lavatories. It is estimated that greywater from a 14-story building can meet the flushing needs of a 30-story building.
However, the lack of public support is the major impedance to the recycling of water in the UAE. Maraqa and Ghoudi (6) conducted a survey to determine the views of UAE nationals concerning the availability of water and their reception of water recycling. Most respondents admitted that there was a likelihood of a water shortage in the future. However, they were only comfortable reusing recycled greywater for open-air activities that did not entail physical contact. On the other hand, very few subjects were willing to install greywater systems in their homes. There was widespread acceptance of reusing recycled greywater for landscape irrigation.
Greywater can be purified through three different means, which can be classified as physical (sedimentation, sorption, and filtration), chemical (precipitation), and biological (plant uptake and microbial decomposition). The choice of treatment method depends on the intended reuse of the recycled water. Treatment methods for indoor reuse of recycled greywater include rotary biological contactors (RBC), membrane bioreactors (MBR), constructed wetlands, and sequencing batch reactors (SBR).
Biofiltration
Biofiltration greywater treatment systems are best suited for the treatment of large quantities of greywater. Biofiltration systems provide an appropriate way of disposing of greywater by addressing the issue of foul odors around treatment plants, preventing eutrophication, and protecting groundwater from pollution.
Constructed Wetland Technology
The constructed wetland technology takes advantage of natural processes to treat wastewater. Their main advantage is their simplicity and relatively low operating costs (Wu et al. 107). A typical constructed wetland system contains plants whose roots have bacteria that biodegrades organic matter in greywater. Wetlands can also be constructed to remove certain nutrients and filter sediments from wastewater.
Constructed wetlands are also used for the purification of greywater meant to be discharged into waterways or to create artificial wildlife habitat. Even though discharging greywater into waterways is not recommended, constructed wetlands help to alleviate the damage. Also, constructed wetlands can be combined with intricate systems to purify water for toilet flushing. Constructed wetland technology has been successfully used in Oman to recycle wastewater from oil factories (Wu et al. 108).
Anaerobic Filtration and UV Disinfection
Anaerobic filtration with UV disinfection has been used successfully in the recycling of greywater in an airport setting. The system yielded recycled greywater of satisfactory quality. Additionally, a financial analysis showed that the initial investment would be recovered within five years (do Couto et al. 377). Dubai airports also employ this method to recycle greywater that is used to wash vehicles. An anaerobic filter made of fiberglass is connected to the greywater treatment unit. Greywater from the collection unit flows into the unit where it is filtered to remove solids as well as organic matter. The water is then disinfected by a UV system.
Reverse Osmosis
Reverse osmosis is a process where a solvent is allowed to pass through a membrane in a direction that is opposite to the concentration gradient. High pressure is often used to elicit the movement of substances against their concentration gradient. Reverse osmosis is widely used in water treatment processes in the UAE to treat water by desalination (Gude 591). The system yields water of very high quality. Reverse osmosis systems can be used together with other physical treatment methods to recycle greywater (Gude 591).
Design/Fabrication/Simulation/Specs Development
This project proposes the use of a physical technology system to treat greywater. Three main steps will be involved the first of which is sand filtration to remove solid particles. The second step will involve membrane separation, particularly, a reverse osmosis system. The third stage will involve disinfection to destroy microbes and ensure that the water meets the required standards for irrigation. A reverse osmosis system will be used alongside UV disinfection. The system will be constructed paying attention to the British Standards for greywater treatment as explained in chapter 3.
Chlorination of treated greywater is among the most commonly used methods of disinfecting greywater. A review of the literature in chapter 2 indicates that excess chlorine has adverse effects on plants when recycled greywater containing high levels of chlorine is used in landscape irrigation. Chlorination systems can also be erratic because their efficiency is dependent on user support to change and add the required chemicals on time. However, given that this project aims at using the recycled greywater for landscape irrigation, alternative means of disinfection should be used to minimize the levels of chlorine in the water while ensuring that the water meets the required standards for irrigation.
UV disinfection offers one of the most effective wastewater disinfection solutions. UV disinfection has several benefits over chlorination, which makes it particularly appropriate for small treatment plants. Chlorination does not require dosage and storage units to hold the disinfectant, which eliminates the need to restock chemicals. UV irradiation gets rid of a wide range of pathogens, including organisms that are resistant to chlorine without producing unnecessary by-products. UV disinfection systems are relatively safe for operators because there is no direct exposure to disinfectants.
The proposed treatment system is an on-site system where greywater will be used again near the population. Therefore, safe reuse can only be attained following the correct treatment that enhances the salubrious, environmental, and visual quality of the water. These requirements lead to the need to offer effective disinfection before reuse. The disinfection system needs to be strong, uncomplicated, safe, cost-effective, and easy to maintain.
The most common problem with operating membranes is fouling, which is the deposition of solutions or solutes on membrane surfaces or pores. Fouling elevates the operation costs of the system because extra processes such as membrane cleaning are required to restore the membrane. Continuing to use a fouled membrane will require elevated operating pressures, a reduction in fluidity, and repeated chemical scrubbing that lowers the lifespan of the membrane.
Various strategies have been proposed to deal with fouling when using membrane systems. Shang et al. (46) noted that there was no fouling when recycling laundry wastewater using an ultrafiltration membrane for 2 hours and 30 minutes at a flux of approximately 130 L.m-2/h followed by reverse osmosis for another 2 hours at a flux of 37 L.m-2/h. A separate study that looked into fouling with membrane systems reported that the likelihood of fouling is proportional to the amount of organic matter present in wastewater, particularly, humic acid concentration (Li et al. 92). Therefore, the best strategy to prevent fouling is to precede the membrane treatment stage by a pretreatment, for example, sand filtration or screening.
Using such an approach on low strength greywater has been reported to lead to residual BOD of 8 mg/L and unnoticeable levels of turbidity and E. coli in the final water, thus implying that the water could meet the most rigorous standards of recycling (Albert et al. 88). Moreover, no membrane fouling was reported. The high level of treatment was attributed to the sequence of steps involved in the treatment. A sand filter served as the pretreatment by getting rid of big particles of organic matter. The membrane and disinfection stages contributed to the rest of the treatment. These findings will direct the design of the proposed greywater treatment system.
Data Collection
It will be necessary to collect different data at various stages of the treatment process. A useful parameter that will need to be noted and recorded is the flow rate of greywater from the source into the pretreatment tank. The BOD of the greywater will also be measured at various points to determine the effectiveness of each step in the treatment process. For example, BOD values of untreated greywater will be recorded in the temporary storage tank, after gravel filtration, after reverse osmosis purification, and after disinfection. Other parameters that will be measured at each of these points include turbidity, pH, COD, and total coliform/100 ml.
Samples will be taken at different times on different days of the week to eliminate bias. The microbial characteristics of untreated greywater will be characterized by 4 species of bacteria: fecal coliforms, S. aureus, P. aeruginosa, and Heterotrophic Plate Count (HPC). Staphylococcus aureus will be cultured on Staphylococcus Medium 110 agar at 35 °C and incubated for 18 to 48 hours. A confirmatory test will be conducted following incubation by performing a coagulation test (Taponen et al. 12). S. aureus will be expected to coagulate the medium due to the presence of coagulase enzyme.
Pseudomonas aeruginosa will be cultured on M-PA-C agar at 41.5 °C for 2 to 3 days. A confirmatory test will be conducted using milk agar. P. aeruginosa breaks down casein in milk to casein to generate yellowish to green coloration. A single streak will be made on milk agar plates and incubated at 35 °C for 24 hours. Colonies displaying a yellowish to green coloration will be counted as P. aeruginosa. The analysis of HPC will be conducted using the spread plate method as described by Duda et al. (393).
Resources Required
The following resources will be required for the construction of the greywater recycling system.
- Pipes to direct greywater from the source into a temporary storage tank.
- A temporary storage tank for the temporary storage of raw greywater before treatment.
- Gravel with different particle sizes for filtration.
- A reverse osmosis system (the Axeon CRO-50 reverse osmosis system).
- A source of power supply.
- A purification tank fitted with ultraviolet lamps for disinfection (a mini-reactor consisting of eight 50W-lamps, low-pressure UV irradiation closed system).
- A water storage tank for the temporary storage of treated water before being used.
- Sand, cement, and ballast to construct the pretreatment unit.
- Labour for the construction process.
Design/Fabrication/Simulation/Specs Development
The pretreatment tank will have a capacity of 20 cubic meters. The filtration chamber, whose capacity will be 18.2 cubic meters, will consist of three layers of gravel: the drainage, transition, and filtration layers as proposed by Laaffat et al. (3). The drainage layer will consist of gravel of particles whose size will range from 15 to 25 mm. The gravel will be layered to a thickness of 30 cm. The transition layer, which will be 15 cm thick, will comprise a gravel of particle sizes ranging from 5 to 15 mm in diameter.
The filtration layer will be 4.1 meters thick and will be made up of particles ranging from 2 to 5 mm in diameter. A connection pipe will link the pretreatment tank to the reverse osmosis system, which will be a commercial high-efficiency reverse osmosis system that is capable of purifying 50 gallons of water per day. A purification tank fitted with ultraviolet lamps for disinfection (a mini-reactor consisting of eight 50W-lamps, low-pressure UV irradiation closed system) will be connected to the reverse osmosis system (Laffaat et al. 3). A schematic representation of the design and layout of the system is indicated below in Figure 3.
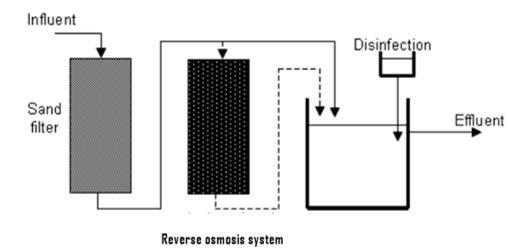
The treated output from the reverse osmosis system will then be disinfected using a mini reactor made up of 8-lamps (50 Watts each) low-pressure UV irradiation closed system (Laffaat et al. 3). The UV device will be an open-channel system consisting of two UV sets. Each set will have four lamps producing monochromatic UV light at a wavelength of 253.7 nm. Dose adjustments will be made by altering the flow rate of the incoming water as well as the number of active banks to attain an effective UV dose of 50 mW/cm2. Sterile bottles will be used to collect samples, which will be taken to the laboratory for various analytical tests to determine the quality of the treated greywater. The disinfected effluent will be stored in a 20 cubic meter tank and used for landscape irrigation.
Expected Results
It is expected that the successful installation of the proposed greywater treatment plant will produce high-quality greywater that meets British standards for recycled greywater use. It is hoped that using recycled greywater for landscape irrigation will improve the overall health of plants in the selected hospitals and hotels. Most importantly, it is anticipated that reusing recycled greywater for landscape irrigation will reduce the clean water demands for these institutions and avail clean water for potable uses.
Project Management
Plan
The proper planning, design, construction, and maintenance of cost-efficient recycled water plants require specific guidelines to ensure that crucial information is not left out. The purpose of the plan is to guide contractors, design architects, engineers, and end-users during the construction, use, and maintenance of the system. A typical wastewater recycling project plan encompasses five stages: the planning, pre-design, design, construction, and maintenance phases.
The planning stage comprises giving precedence to possible recycled water connections, documenting each site into a tracking database, and creating public awareness of the recycled water. In the pre-design phase, crucial information that guides the design of the project is gathered. Additionally, there is a need to liaise with the site representative to introduce the concept of recycled water use, carry out site visits, utility research, and an analysis of the soil sample.
The design phase entails the development of the construction plans and specifications according to the relevant system and design standards. These plans need to be approved before construction. The construction phase, on the other hand, encompasses the building of the recycled water system, assessment of the system, conducting field tests, performing the final inspection, and linking the system to the recycled water supply. The maintenance phase, which is the last stage, involves the guidance of workers responsible for servicing the system towards the proper use and maintenance of the recycled water system. Other aspects include using best administration practices to minimize surface runoff and stagnation of recycled water as well as conducting yearly site inspections of the project. Details of each step are provided in the following subsections.
The Planning Phase
The planning phase of a recycled water system begins with prioritizing potential recycled water connections to identify the most cost-effective use of recycled water on the proposed sites. When screening each hotel and hospital site to determine the most appropriate sites, some of the factors that will be taken into consideration include finding out the yearly demand for irrigation water from the irrigation meter accounts and the approximate cost of establishing the greywater treatment plan relative to the cost of obtaining clean water for irrigation.
It will also be necessary to determine the cost of connecting the proposed greywater treatment system to the existing water network, the right of way requirements, and planned expansions or reductions. Expansions and reductions may affect the demand and supply of treated and untreated greywater respectively. The proposed use of recycled greywater will also be considered. For instance, the use of clean water will be sustained in areas set aside for small children, for example, playgrounds.
Additionally, adequate measures will be taken to separate potable and greywater systems in those areas. Sections containing artificial turf will only use potable water to cool off the surface of the grass to avoid ponding of recycled greywater. These criteria will enable the assessment of the overall cost per acre or foot. Benefit per acre or foot will also be determined, after which a comparison of the cost versus benefit of each site will identify suitable sites for the greywater treatment project.
A tracking database will be made to keep track of all sites. A basic framework that will be used to develop the database is the Microsoft Access database. This database will facilitate the tracking of the projectâs status at each site. The principal investigator will appoint project managers at each of the selected sites during this phase.
Public outreach and education of the hotel and hospital staff and administrators will be necessary for the successful execution of the greywater recycling system. The design architect will prepare a letter inviting the staff members and administrators to a public outreach meeting. Two possible dates will be included in the letter as well as a telephone number that the recipients can use for questions. Material for the public outreach meeting will be developed and reviewed in readiness for the event. Information regarding water supply challenges, the impact of climate change on water resources, and the proper use of recycled greywater will be included in the outreach material. During the outreach, the design architect will respond to all concerns raised by the public about the use of recycled greywater for landscape irrigation.
Pre-Design Phase
In the pre-design phase, crucial information that guides the design of the project is gathered. Additionally, there is a need to liaise with the site representative to introduce the concept of recycled water use, carry out site visits, utility investigation, and an analysis of the soil sample. The design architect will assist the principal investigator to coordinate with the site supervisors. A contact letter indicating details of the recycled greywater project, objectives, and requirements will be written to the identified sites.
Thereafter, the design architect will obtain the sitesâ as-built sketches for the clean water and irrigation water systems. Initial site visits will be conducted to familiarize the design architect with the sites. During the site visits, the architect will be able to identify any design challenges and factor them in the development of the system.
Usability research (also referred to as utility research) is a crucial stage of the pre-design phase. The purpose of this process is to identify underground utilities and factor them in alignment inquiries to minimize possible disagreements. The operations of all existing utilities should not be interrupted by the new project. Relevant permits should be obtained if recycled water pipelines need to be set up in a prevailing utility easement. Therefore, the utility drawings should be recorded and indicated on the design plans. The engineer will be responsible for obtaining the required permits.
Soil sampling and analysis will be conducted to encourage healthy plant growth and inhibit erosion and excessive overflow. Soil analysis data will also inform the use of organic matter in different areas of the landscaped areas as required. Imported soil and fill materials will also undergo extensive testing. Soil sampling will also ensure that the fill materials are safe for the workers, patients, and clientele of the institutions. A soils engineer will test the soil for grading and compaction among other engineering parameters, whereas a certified environmental specialist will check for biological, chemical, and environmental aspects of soil.
The Design Phase
The design phase entails the development of the construction plans and specifications according to the relevant system and design standards. These plans need to be approved before construction. Therefore, it is important to understand the local and state regulations and paradigms of the proper design of a recycled greywater system and use them all through the design process. The design plans will consider all the details regarding British standards of greywater recycling as indicated in chapter 2.
The recycled water system will operate independently of all clean water systems in the facilities. Any form of cross-connections between the systems is forbidden. For sites that will use clean water and recycled greywater for irrigation, distinct irrigation controllers will be installed for each type of water. One design requirement is that all irrigation lines projecting above the ground surface will be cut and covered below the surface.
All sources of drinking water will be safeguarded from direct contact with recycled water. Also, pipelines conveying clean water and recycled greywater will be separated from each other at specified minimum distances. The design architect will meet with the relevant water management bodies, utility agents, government organizations, and business owners to obtain applicable permits and documents that will be part of the construction documents. The architect will also meet with the contractor, present the plan, and agree on a specified budget to execute the project. The budget will include the cost of all irrigation components and labor estimates.
The Construction Phase
The construction phase comprises building the greywater recycling water system, assessing the system, conducting field tests, performing the final inspection, and linking the system to the recycled water supply. The contractor will work together with the site project manager, during the construction phase of the project. The contractor will be tasked with the duty of exploring all potential construction methods that can be used in the project and evaluating the possible impact of using certain methods in specified areas. For example, trenchless operations are more suited for areas with major roads than in areas with minor roads.
Therefore, the construction methods will be chosen to lessen the effect on traffic and utilities such as storm drains with no significant increases in the overall construction costs. Feasibility studies of the different construction methods will be conducted in the initial stages of the construction phase to find the most practical and cost-effective techniques. The contractor will also keep track of to-do lists and finances of the project. Other roles of the contractor during this stage include updating the schedule, providing progress reports of the completed work, documenting project matters, noting down the state of the project, and ensuring that it aligns with the financial plan.
A crucial component of operating a greywater recycling system is avoiding cross-connections (Oh et al. 20). The contractor will set up the cross-connection control test by enlisting the services of an authorized specialist in the procedure. The cross-connection control test serves to confirm that the recycled water system does not have any links with the clean water system. The cross-connection test is usually performed after the construction work is over but before joining the irrigation system to the recycled water system (Kehoe and Scarpulla 731).
The cross-connection test will be done by noting down and keeping an eye on the pressures of the recycled and potable water systems over two hours. An increase in pressure in either system will be an indication that cross-connection may be present. On the other hand, steady declines in pressure are indications of probable leaks in the systems. The last inspection procedure will entail exposing the clean water pipes for scrutiny to make certain that no cross-connections exist. The project sketches will then be revised to as-built plans following the approval of the site and connection of the plumbing system to the greywater recycling system.
The Maintenance Phase
The maintenance phase will entail the training of workers and end-users towards the responsible and lawful use of the greywater treatment system. Other aspects include using best administration practices to minimize surface runoff and stagnation of recycled water as well as conducting yearly site inspections of the project. The training material for staff members will consider the following information: an overview of recycled water, production aspects of recycled greywater, the quality requirements of recycled water, as well as the rules and regulations governing the production and use of recycled greywater. Safety aspects about cross-connections and prevention of backflow will also be part of the training.
All man-made systems are prone to error and greywater recycling systems are no exceptions. Therefore, it is important to set up an emergency response plan for the system. In cases of suspected cross-connection, immediate shut down of recycled and clean water supplies will be required. Samples will be drawn from the potable water sources and subjected to bacteriological testing. Troubleshooting will be done to identify and eliminate the point of cross-connection.
The clean water pipe system will undergo rigorous disinfection using a concentrated chlorine solution (Sharvelle et al. 3801). A minimum residual chlorine concentration of 50 parts per million (ppm) will be left in the drains for at least 24 hours, after which the system will be cleaned out and additional tests performed on the drinking water (Besmer et al. 1900). The reactivation of the clean and recycled water systems will only be approved if the test results are satisfactory.
Routine maintenance of the recycled greywater system will be conducted to comply with the local set of laws and ensure the integrity of the system. Sprinkler heads will be adjusted to attain the required head-to-head coverage all through the intended arcs (Miller et al. 137). Care will be taken to ensure uninhibited rotation of sprinklers and eliminate blockages. Pressure regulators will be checked to ascertain that the system is operating at the recommended pressure. Occasionally, a pressure gauge will be used to confirm the pressure readings. The precision of time clocks will be checked and standardized appropriately using a stopwatch.
All broken components (valves or sprinklers) will be replaced or repaired as soon as they are noted. System uniformity will be ensured by replacing broken components with the right model numbers. Backflow deterrence gadgets, pumps, and other accessories will be checked regularly for seepages and other defects that may compromise the functioning of the irrigation system. Screens and backwash filters will also be checked regularly.
Programme of Work
Table 6 indicates the work program for the planning and execution of the greywater recycling project.
Table 6. Program of Work.
Responsibility Matrix
Different individuals have varying roles throughout these phases. All the involved parties must understand their roles in the development of the water-recycling project. A summary of the roles and responsibilities of each party is provided in the responsibility matrix in table 7.
Table 7. Responsibility Matrix for the Implementation of a Greywater Recycling Project for Landscape Irrigation.
Quality Assurance
Quality assurance and quality control are vital to the monitoring phase of the project because they foresee and help to avoid certain errors. Quality assurance encompasses managerial actions such as assigning duties and responsibilities to the stakeholders of the projects, training of staff, and authentication of data. It also involves conducting reviews of laboratory findings and taking the necessary measures.
Quality control, on the other hand, is the enactment of processes to enhance the veracity of monitoring data. Useful aspects of quality control include techniques for the appropriate collection, treatment, and storage of samples, duplicate sampling, scrutiny, and standardization of equipment to ensure data accuracy. All projects should prepare quality assurance and quality control protocols and use them for each phase of the monitoring program.
Meaningful data can only be available if the samples are obtained from the right place using the correct procedures by trained personnel. Poor-quality sample collection procedures and testing methods can yield false positive and false negative outcomes thus misguiding the decisions that depend on these data. Principles of reliable monitoring necessitate that the particulars of monitoring are expressed unambiguously, including the aspects to be monitored, where the monitoring should take place, and who should conduct the monitoring.
Itemized standard operating procedures will be written down so that the process can be reproduced by an independent party should the need arise. The monitoring techniques will be based on industry paradigms to guarantee the consistency of the resultant data with other datasets. The consistency of data also requires that only certified suppliers of raw materials and machinery are used in the design, construction, and monitoring of the greywater system.
All personnel who will handle the system will undergo adequate training for the use of the system as well as the monitoring process. Since training is an unending process, the training material will be appraised frequently to make certain that the stakeholders uphold the right qualifications. It will also be necessary to confirm the competence of the operations workforce, especially for activities that have a substantial effect on the quality of recycled greywater.
It is the role of the project managers at each site to ensure that the on-site greywater recycling system is used by the system specifications. The project managers also need to acquaint themselves with the type of system installed, its location, and performance. Therefore, an appropriate and consistent maintenance and monitoring program is needed to guarantee the correct operation of the greywater recycling unit. Some of the measures that will be put in place to attain a quality controlled treated greywater system include enforcing pollution cutback strategies such as managing of trade waste, consistent treatment procedures, monitoring the efficiency of the disinfection systems, and locking up the dissemination systems.
The premises will also recruit competent and skilled operatives and custodians. Also, there will be a continuous enhancement of performance and maintenance initiatives. The premises will also keep up with the review, monitoring, and reporting programs. Another crucial point is having emergency strategies for unforeseen system failures. To ensure optimal performance of the system, samples will be collected and tested as indicated in table 8. During the authorizing phase of the project, a large number of samples will be taken and tested regularly (every week). The frequency of sampling and testing will reduce as indicated in table 8 following three months of operation.
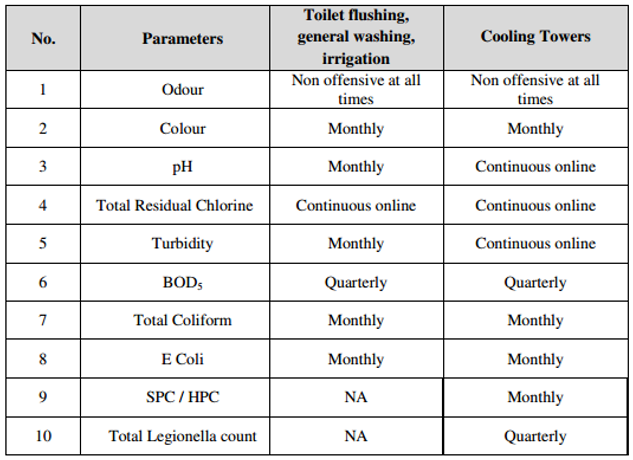
Water samples need to be taken from points that best represent the status of the entire system. In cases where more than one tank is in use, samples will be collected from the upstream storing reservoir to assess the condition of the purified water. The rationale for this sampling is that any ensuing reservoirs are likely to be disturbed by fluctuations in temperature or mixed with water from the standby water source.
All workers concerned with the operation of the greywater system will undergo the required training to perform their duties efficiently. They include contractors, operatives, and other workers whose actions have a significant impact on the quality of greywater as well as public wellbeing. End users also require training, particularly in situations where end-user constraints apply. Contractors, operators, and end-users will have a good knowledge background to facilitate the making of sound operational decisions. These people need to understand the consequences of their actions on the quality of greywater.
For instance, treatment plant workers should grasp the idea of water treatment and know the rationale for every action in the treatment process. Such knowledge will help them to react appropriately to fluctuations in the quality of greywater by modifying the treatment process as needed. Farmers (in this case, landscape artists) will need to have an in-depth understanding of the soil parameters and its need for fertilizers and other soil additives. Therefore, the level of skills and training should match the knowledge levels expected of the system operatives, contractors, and end-users. A quality assurance strategy concerning training is identifying the training requirements and making certain that adequate resources are obtainable to sustain relevant programs.
Training for end-users will consider clarifying end-user limits as well as best management routines to ensure that the users comply with these restrictions. The rationale for the restrictions will also be provided by clarifying the health and environmental consequences of failing to abide by the limits. Examples of pertinent areas to tackle include general information such as the overall quality of water, the chemical and microbiological aspects of water, and the differences between the chemistry of soil and groundwater.
Detailed training to augment the performance of the recycled greywater system for contractors and operators will include information on the basics of recycled water treatment, for example, primary, secondary, and tertiary treatment. Aspects of irrigation management for various uses and plumbing codes used in recycled water systems will be emphasized. Water treatment aspects such as on-site purification of greywater, workings of filtration plants and disinfection systems, and the dissemination of treated wastewater will be taught. Quality control and quality assurance practices such as a collection of samples, analysis of treated greywater, construal, and logging of results, in addition to maintenance of equipment will also be included in the training.
Particular training areas for end-users will include information on the correct use of recycled greywater, storing recycled greywater, environmental threats posed by greywater, management of nutrients and fertilizer when using greywater for irrigation, controlling salinity in the course of greywater use, as well as scheduling of irrigation. Other aspects that will be considered include the identification and control of algae, handling drainage and overflow issues, good health and safety practices, and reporting of greywater issues. Operators, contractors, and end-users will also receive guidance on the management of greywater quality in the areas of reaction to emergencies, certification, record keeping, recounting, and research and development.
The training will employ various approaches, for example, the provision of official training courses recognized by a national training organization, especially for the contractors and greywater system operatives. In-house training and on-the-job experience will also come in handy for the operators, especially when troubleshooting greywater quality issues after the system has been commissioned. Workshops, seminars, and short refresher courses will also be organized regularly. To ensure that all end-users receive the required information, brochures, manuals, and newsletters will be prepared and distributed.
Documentation
All data collected from the various tests at different times will be documented manually on paper. However, for long-term storage of data, the paper records will be converted into soft copies. The training process will also be documented as recommended by Burgess et al. (570). Records of all contractors, operatives, and target users who will take part in the training will be kept. Strategies for assessing the effectiveness of the training will also be determined and recorded. Reporting of data will provide sufficient information regarding the techniques used and indicate the extent of certainty in the approximations provided with the results. Additional details regarding documentation and record-keeping are included in section 5.3.
Information
The specific information that will be collected and recorded will include physicochemical attributes of greywater such as pH, BOD5, and COD. These data will be taken once every five days. At least 20 samples will be taken for each test. Microbiological parameters will be checked every two weeks. Samples will be stored in dark glass bottles at 4 oC before analysis.
Record Keeping
Accurate and timely record-keeping procedures facilitate the quality provision of services. There is a need to keep accurate and up-to-date data regarding the operations of the greywater treatment plant. The described quality control procedures will be documented and kept for future reference. The project manager at each site will be responsible for keeping these documents. However, copies of the standard operating procedures used to run the system will be accessible to all employees of the facility who will be involved in operating the wastewater system.
Checks and Reviews
The property owner and the greywater recycling system operative will enforce a scheduled monitoring and performance-recording program after the commissioning of the system. The goal of the collected data is to ascertain that the quality of the treated greywater is fit for the intended use. This process also makes it possible to identify and rectify any potential problems that may arise in the course of use. Certain measures need to be taken to ensure that remedial action is taken promptly.
The flow of raw greywater, as well as the flow of the treated water, needs to be checked regularly. The quality of the treated greywater will be checked at the system outlet. Finally, the disinfection system will also be checked. For chlorination systems, this process would mean quantifying chlorine used in the tank and replenishing it appropriately. However, for UV irradiation systems, the quality of the UV lamps will be checked and any faulty lamps will be replaced promptly.
Apart from monitoring the quality of treated greywater, other components of the system will also be checked. These include membranes, filters, biological, and disinfection units. Other elements such as pumps, control units, pump controls, and backwash will be checked and maintained as per the manufacturersâ instructions. Residual organics may continue growing in dead spots despite adequate disinfection. Such growth may cause the blockage of pipes and foul smells.
As a result, it may be necessary to drain the pipes with clean water to lower the chances of contamination. Monitoring will also involve regular checks to ascertain the integrity of the membrane. These checks will be conducted according to the manufacturersâ instructions. The assistance of a certified service contractor will be sought to help with the maintenance of the greywater system.
Irregularities in the quality of treated greywater will be noted down in records that will be kept by the owners of the premises. Sampling and testing of treated water will also be done following reports of waterborne illnesses. If treated greywater fails to meet the required standards, it will be emptied into the sewage system. If subsequent batches of greywater contain bacteriological strictures that surpass the guideline values, the use of the system will be suspended until the problem is identified and rectified. Additional tests will be conducted for parameters that fail to meet the required standards after which remedial measures will be taken. Thereafter, a sample will be selected at each sampling spot and retested to confirm the effectiveness of the remedial measures.
Revision Recording
All changes that will be made to the greywater system through the monitoring or maintenance processes will be updated on the design plans for the system. However, the original as-built designs will be retained. The standard operating procedures associated with the faulty outcomes will be amended and used for subsequent quality assurance and quality control efforts.
Works Cited
Albalawneh, Abeer, and Tsun-Kuo Chang. âReview of the Greywater and Proposed Greywater Recycling Scheme for Agricultural Irrigation Reuses.â International Journal of ResearchâGranthaalayah, vol. 3, no. 12, 2015, pp. 16-35.
Albert, Ryan J., et al. âFeasibility of Potable Water Generators to Meet Vessel Numeric Ballast Water Discharge Limits.â Marine Pollution Bulletin, vol. 120, no. 1-2, 2017, pp. 82-89.
Besmer, Michael D., et al. âLaboratory-Scale Simulation and Real-Time Tracking of a Microbial Contamination Event and Subsequent Shock-Chlorination in Drinking Water.â Frontiers in Microbiology, vol. 8, 2017, p. 1900.
Bichai, Françoise, and Nicholas Ashbolt. âPublic Health and Water Quality Management in Low-Exposure Stormwater Schemes: A Critical Review of Regulatory Frameworks and Path Forward.â Sustainable Cities and Society, vol. 28, 2017, pp. 453-465.
Burgess, Jo, et al. âInternational Research Agency Perspectives on Potable Water Reuse.â Environmental Science: Water Research & Technology, vol. 1, no. 5, 2015, pp. 563-580.
Cassini, A., et al. âImpact of Food and Water-Borne Diseases on European Population Health.â Current Opinion in Food Science, vol. 12, 2016, pp. 21-29.
Cook, Christina. âRegulating the Risks of Domestic Greywater Reuse: A Comparison of England and California.â Built Environment, vol. 42, no. 2, 2016, pp. 230-242.
do Couto, Eduardo de Aguiar, et al. “Greywater Treatment in Airports Using Anaerobic Filter Followed By UV Disinfection: An Efficient and Low-Cost Alternative.” Journal of Cleaner Production, vol. 106, 2015, pp. 372-379.
Duda, Scott, et al. âLack of Correlation between Legionella Colonization and Microbial Population Quantification Using Heterotrophic Plate Count and Adenosine Triphosphate Bioluminescence Measurement.â Environmental Monitoring and Assessment, vol. 187, no. 7, 2015, p. 393.
Fountoulakis, M. S., et al. âSingle House On-Site Greywater Treatment Using a Submerged Membrane Bioreactor for Toilet Flushing.â Science of the Total Environment, vol. 551, 2016, pp. 706-711.
Giusti, L., and M. Almoosawi. âImpact of Building Characteristics and Occupantsâ Behaviour on the Electricity Consumption of Households in Abu Dhabi (UAE).â Energy and Buildings, vol. 151, 2017, pp. 534-547.
Giwa, Adewale, and Abdallah Dindi. âAn Investigation of the Feasibility of Proposed Solutions for Water Sustainability and Security in Water-Stressed Environment.â Journal of Cleaner Production, vol. 165, 2017, pp. 721-733.
Gosling, Simon N., and Nigel W. Arnell. âA Global Assessment of the Impact of Climate Change on Water Scarcity.â Climatic Change, vol. 134, no. 3, 2016, pp. 371-385.
Green, Pamela A., et al., âFreshwater Ecosystem Services Supporting Humans: Pivoting From Water Crisis to Water Solutions.â Global Environmental Change, vol. 34, 2015, pp. 108-118.
Gude, Veera Gnaneswar. âDesalination and Water Reuse to Address Global Water Scarcity.â Reviews in Environmental Science and Bio/Technology, vol. 16, no. 4, 2017, pp. 591-609.
Kehoe, Paula, Sarah Rhodes, and John Scarpulla. âRe-Thinking Water Sources: Regulatory Blueprints for Non-Potable Water Use.â Proceedings of the Water Environment Federation, vol. 2014, no. 11, 2014, pp. 730-743.
Laaffat, J., et al. âBiotechnological Approach of Greywater Treatment and Reuse for Landscape Irrigation in Small Communities.â Saudi Journal of Biological Sciences, 2017, pp. 1-8. Web.
Leong, Janet Yip Cheng, et al. âProspects of Hybrid Rainwater-Greywater Decentralised System for Water Recycling and Reuse: A Review.â Journal of Cleaner Production, vol. 142, 2017, pp. 3014-3027.
Li, Kai, et al. âPerformance of Adsorption Pretreatment in Mitigating Humic Acid Fouling of Ultrafiltration Membrane under Environmentally Relevant Ionic Conditions.â Desalination, vol. 377, no. 2016, pp. 91-98.
Maraqa, Munjed A., and Kilani Ghoudi. âPublic Perception of Water Conservation, Reclamation and Greywater Use in the United Arab Emirates.â International Proceedings of Chemical, Biological and Environmental Engineering, vol. 91, 2015, pp. 24-30.
Miller, Grady L., et al. âGolf Course Irrigation Systems’ Distribution Uniformity Affects Soil Moisture Variability.â European Journal of Horticultural Science, vol. 79, no. 3, 2014, pp. 135-141.
Mlih, Rawan, et al. âSoil Organic Matter Amendments in Date Palm Groves of the Middle Eastern and North African Region: A Mini-Review.â Journal of Arid Land, vol. 8, no. 1, 2016, pp. 77-92.
Oh, Kai Siang, et al. âA Review of Greywater Recycling Related Issues: Challenges and Future Prospects in Malaysia.â Journal of Cleaner Production, vol. 171, 2018, pp. 17-29.
Public Utilities Board. Technical Guide for Greywater Recycling System. 2014. Web.
Shang, Xia, et al. âCoagulation Strategies to Decrease Fouling and Increase Critical Flux and Contaminant Removal in Microfiltration of Laundry Wastewater.â Separation and Purification Technology, vol. 147, 2015, pp. 44-50.
Sharvelle, Sybil, et al. âRisk-Based Framework for the Development of Public Health Guidance for Decentralized Non-Potable Water Systems.â Proceedings of the Water Environment Federation, vol. 2017, no. 8, 2017, pp. 3799-3809.
Taponen, Suvi, et al. âSpecies Distribution and In Vitro Antimicrobial Susceptibility of Coagulase-Negative Staphylococci Isolated From Bovine Mastitic Milk.â Acta Veterinaria Scandinavica, vol. 58, no. 1, 2015, p. 12.
âWater Recycling and Reuse.â United States Environmental Protection Agency (US EPA). 2017. Web.
Wu, Shubiao, et al. âTreatment of Industrial Effluents in Constructed Wetlands: Challenges, Operational Strategies and Overall Performance.â Environmental Pollution, vol. 201, 2015, pp. 107-120.