This section aims to review previous studies that relate to plasma-laser radiation interaction during dual-pulse laser ablation of metals and alloys. To ensure a detailed review of the studies, the section discusses laser-induced breakdown spectroscopy, laser ablation, and atomic emission spectroscopy. Additionally, figures and graphs are included to ensure an in-depth review.
Laser-Induced Breakdown Spectroscopy
Laser-induced breakdown spectroscopy (LIBS) is quick chemical analysis technology that creates a micro-plasma on the sample surface using a brief laser pulse. When LIBS is compared to other elemental analysis methods, it has numerous advantages. Li et al. review signal enhancement methods in LIBS (2). Further on, the study reveals that LIBS is limited by low sensitivity and high detection limits compared to alternative analytical techniques such as inductively coupled plasma mass spectrometry. For the past 20 years, different methodologies have been used by LIBS to ensure extreme quantitative and qualitative analysis is achieved. Some of the methodologies being used right now are spatial confinement, double-pulse excitation, and spark discharge. To realize these improved methodologies, numerous configurations of experimental setup and conditions are recommended while different parameters substantially impact the improvement effect. Numerous parameters like plasma volume and emission intensity have been reviewed by Li et al. to ensure studying of the laser ablation procedure and characterize the method’s effectiveness. Several theories are presented to explain how each improvement method works. These modified procedures have been used on a variety of materials and in a variety of fields.
The short-duration pulsed laser beam used in LIBS usually focuses on the sample used to ensure it evaporates. Further on, the vapor is subsequently atomized and ionized by the pulsed laser beam to result in a plasma that excites atoms and ions (Figure 1). When expansion and cooling of plasma happens, atoms and ions relax to low-energy states by producing particular radiations underpinning electronic transitions (Figure 2). A spectrometer in the UV visible-NIR region is then used to determine the visible signal associated with plasma production. The observed atomic lines’ wavelengths provide qualitative information on the existence of elements within the laser-induced plasma and, as a result, on the sample (Fabre 2). Finally, the intensities of the lines are related to the element concentrations and the plasma properties.
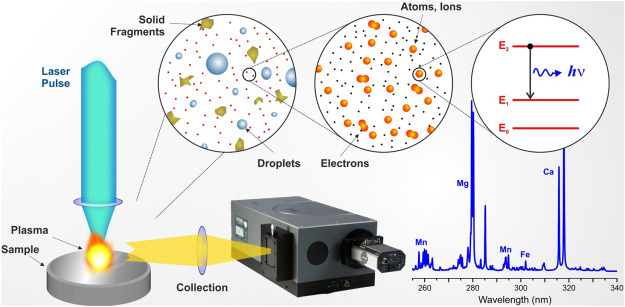
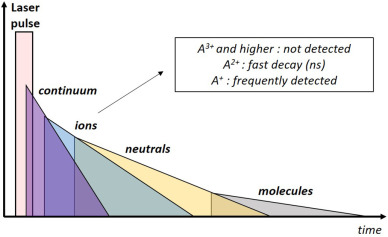
Numerous quantitative and qualitative studies have been done using calibrated samples while monitoring emission line locations and concentrations. In 2020, the LIBS technology was used on around 200 universities on five continents and one interplanetary sensor on Mars. LIBS analysis performed in the study seems unlimited with optical equipment, remotely, and under diverse environmental circumstances (Fabre 3). Additionally, over 5000 publications about LIBS technology have been published within the last five years, ranging from fundamentals to application (Dubey et al. 3). These two studies show that LIBS technology is increasing because it is used for publications and conducting different types of research.
Recent technological advancements have increased the application of the LIBS method. Some notable advancements include the emergence of CCD in the 1990s and the development of compact CCD in the 2000s (Dubey et al. 7). These advancements have helped LIBS imaging develop and introduce new laser sources in the last decade. Botto et al. claim that LIBS has dominated the analytical atomic spectroscopy landscape for the past decade. This is similar to atomic absorption spectroscopy that ruled the 1960s and 1970s, ICP atomic emission spectroscopy domination in the 1980s, and ICP mass spectroscopy in the 1990s. Therefore, LIBS is a highly appealing technology that stands out for its countertop and remote instrumentation. LIBS has a speed of operations, abilities to operate in an ambient environment, and the capacity to produce megapixel multi-elemental images with micrometric resolution and ppm-scale sensitivity (Figure 3).
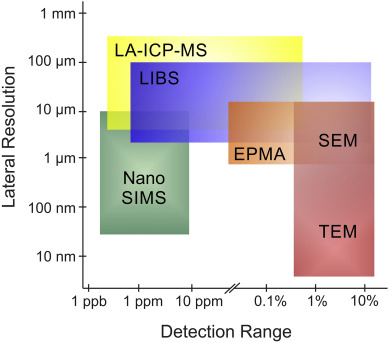
Although LIBS has been largely applied to topics such as biology, industrial applications, plasma investigation, and archeology, it has mostly impacted earth science. Further than the LIBS setup’s relative simplicity, geologists are interested in the LIBS approach because of its capability to excite a variety of sample types with minimal sample pretreatment. In 2018, geology publications increased from a few articles recorded a decade ago to over 20. Fontana et al. study; summarized the most recent works on geochemical and geological LIBS technologies. Additionally, they considered the planetary LIBS application, which uses the ChemCam instrument onboard the Curiosity rover on Mars, to broaden their scientific audience. Generally, LIBS has significantly impacted earth sciences through a series of publications and studies.
In most cases, the double pulse is primarily used when quantitatively studying many different items, including tooling made of multicomponent alloys. Ermalitskaia and Voropay claim that double pulse has advantages such as no chemical needed for pretreatment on the surface and a low degree of sample destruction (1). Due to these benefits, LIBS is one of the most common direct analysis techniques for thin protective coatings, which significantly enhance the functionality of the products and increase their durability and resistance to wear. However, few articles focus on LIBS of thin layers on commercial samples, whereas the literature has extensively examined and adequately documented quantitative analysis of massive objects made of multicomponent alloys. This is why Ermalitskaia and Voropay conducted a study focusing on titanium-based PVD coatings with double pulse LIBS and submicron resolution.
PVD coatings are usually coated on the drilling and cutting tools which frequently have incredibly complicated geometries. For Ermalitskaia and Voropay to realize quality control in their study, they performed a flat blank sample, whose coating can be examined under an electron microscope and using X-ray spectroscopy similar to PVD-coating. Unfortunately, there are specific issues with the element concentration control in the tool’s layers at various locations along the cutting rim and the uniformity of the deposited layer on the drill’s complicated surface. A submicron-resolution examination of multilayer coatings on the curved surface cannot be done using conventional techniques. However, their main goals are to investigate the opportunities provided by direct layer-by-layer examination of PVD coatings and develop methods for the submicron analysis of coatings placed on diverse objects using double-pulse laser atomic-emission spectroscopy were achieved.
Laser Ablation
The technique of removing material from a solid surface by irradiating it with a laser beam is known as laser ablation. Nguendon Kenhagho et al. argue that when a laser irradiates the targeted surface, numerous processes include transmission, reflection, absorption, and dispersion (Figure 4). The figure represents incident, absorbed, reflected and transmitted energy represented by P0, Pβ, PR, and Pζ. Further, the ablation phenomenon occurs when the laser energy absorbed by the target reaches a specified value (ablation threshold). However, long-wavelength lasers are not appropriate for metal ablation because the metal surface reflects most of the laser energy. Metal ablation can be accomplished with UV short-pulsed lasers with short wavelengths, such as a frequency multiplied Nd: YAG laser or an excimer laser (Zhang et al. 530). An infrared laser with a long wavelength can be effective when ablating some particular materials.
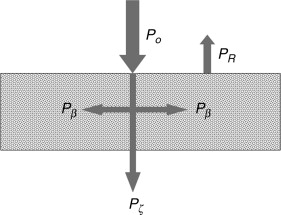
During laser ablation, a unique interaction between radiation and matter is influenced by laser parameters such as irradiation spot radius, output power, and wavelength. When irradiated with a low power density laser, the target under irradiation will heat up. The target is ablated when the power density surpasses the ablation threshold energy at which evaporation begins at the surface (Zhang et al. 541). Whenever a pulsed laser is used to irradiate a solid target, most of the laser energy is stored on the nontransparent object’s surface (Nguendon Kenhagho et al. 1338). Since this thin layer absorbs a portion of the laser light beneath the irradiated surface, the average temperature continues to rise. Additionally, a portion of the energy is carried into the internal lining at the same time. As a result of energy, the heated region’s thickness grows. The temperature gradient gets narrower as the thickness increases, resulting in a progressive drop in heat conduction rate until it reaches zero. Just a tiny target layer can be penetrated by laser heat energy. The thermal penetration depth is the thickness of this thin layer.
Studies on high-density and high-temperature plasmas have been created by focusing high peak power laser pulses onto a destination. Lee et al. emphasize putting laser processes and their basics as physical mechanisms (4). This emphasis aims to ensure that main experimental techniques such as mass spectrometry, optical emission, and absorption spectroscopy are devised to characterize laser-produced plasma. The fundamental theoretical and numerical methodologies used by Lee et al. ensure laser-target interaction and plasma production (7). The study compares and references results from multiphase targets, despite its major focus on metal target ablation.
Since early 2000, institutions have been using ultra-short double pulse in lasing imaging has gained popularity. In the last decade, micromachining has been invaded by pulse bursts made up of multiple pulses with a delay of a few tens of nanoseconds or less. This invasion has completely opened up new process regimes to increase framework rates and machined samples’ surface quality. Additionally, new knowledge of the relevant machining tactics and processing regimes has emerged due to numerous physical factors such as shielding and material re-deposition. During laser cutting, experimental and numerical research results are put into context for various time scales. Förster et al. focus on the underlying physical events that occur during burst processing and their influence on metal cutting outcomes in the ultra-short pulse domain, with latencies varying from a few hundred fs to several nanoseconds (9).
Despite the fact that laser ablation in the liquid phase (LAL) has been around since the 1990s, laser colloids synthesis has gained prominence in the previous decade due to considerable advances in production rate. Due to improvement, LAL has demonstrated comparative advantages in biomedical and catalysis applications and recent commercialization. However, because the process relies on highly transitory events, the LAL synthesizing refining study lacks real knowledge. Experimental and theoretical investigations have been done to provide a fundamental understanding of the underlying procedures due to the complexity of the physics and chemistry involved. However, temporal and spatial resolution and non-equilibrium conditions have hampered these efforts (Nguendon Kenhagho et al. 8). The procedures occurring at the earliest time scales, ranging from femtoseconds to several microseconds, appear to be crucial in defining the outcome.
Atomic Emission Spectroscopy
Atomic emission spectroscopy (AES) is a chemical analysis technique that employs light intensity produced at a certain wavelength from a flame to quantify an element in a sample. The element’s identification is determined by the frequency of the atomic spectral line, while the intensity of the emitted light is correlated to the number of atoms in the component. Atomic emission spectroscopy studies the emission of radiation from analytic atomic vapors that have been sufficiently stimulated (Ren How et al. 5). Additionally, the emitted photon and its strength are the foundation for the technology’s qualitative and quantitative implementations. It’s a multi-element analytical approach that can be used to examine gaseous, liquid, powdered, or solid substances. It is the most often used method for analysis due to its high detection power and wide range of excitation sources (Botto et al. 85). An inferential coupled plasma (ICP) works as an atomization excitation source among the atomic emission spectroscopy; the approach is known as ICP-availability of sufficiently sensitive interfering free spectrum data.
As a non-invasive plasma diagnostic tool, atomic emission spectroscopy was used to detect light emission during titanium nitride deposition using magnetron sputtering plasma discharge. Ren How et al. claim that during the discharge, the atomic emission of argon and titanium was employed to detect the ionization ratio of each element (8). Investigation of the ionization mechanism of titanium atoms is highly significant during the deposition of titanium nitride (TiN) thin film employing reactive magnetron sputtering plasma using ionized physical vapor deposition (IPVD) technology. Further on, the study uses a simple formula: the atomic emission of nitrogen spectrum to calculate gas rotational temperature, which is then assessed under various discharge conditions. The study compares two alternative spectrometer resolutions for the estimation of gas temperature. Even when employing a low-resolution spectrometer, the measured temperatures were nearly identical to those expected in high-pressure magnetron sputtering plasmas.
Plasmas, among the Power-to-X technologies, have a lot of promise for optimal use of intermittent renewable energy sources. High CO2 conversion efficiencies have been recorded using electromagnetic plasmas under vacuum conditions; however, these are not suited for CO2 reduction on a large scale (Fabre 9). Researchers show that ultrafast pulsing of microwaves permits significant increases in energy efficiency during CO2 splitting at atmospheric pressure when compared to continuous-wave operation of the microwave source. In addition, the vibrational and rotational temperatures were tracked throughout time. Identify a time frame where non-equilibrium may be expected at the beginning of the pulse of an atmospheric CO2 microwave plasma using time-resolved optical emission spectroscopy for the first time (Botto et al. 86). Despite the indications of non-equilibrium in the study, the CO2 dissociation was dominated by the thermal mechanism. The study discovered that precision control of the transfer of energy in the plasma may be achieved with rapid pulse of the microwave power generation.
Various mechanical qualities are required to suit the designer’s requirements in many industrial applications, especially suspension systems. Dubey et al. use LIBS calibration curves, a semi-nondestructive approach employed to determine the hardness (HV units) of the light-duty low-carbon spring steel DIN50Cr3 (Botto et al. 100). Three different spring steel samples, DIN50Cr3, were effectively treated for this purpose by undergoing several treatment regimens, including annealing (R1), quenching (R2), and normalizing (R3). The calibration curves in the study were created by plotting the ratio of ionic to atomic line intensities in LIBS spectra against Vickers mechanical hardness and performing microstructural investigations (Figure 5). The physical hardness of spring steel DIN50Cr3 subjected to various tempering heat treatment regimens R4, R5, and R6 is then calculated using the calibration curves. The results are compared to those acquired using the Vickers test (Fontana et al. 312). A microstructural study was carried out using scanning electron microscopy (SEM). The variation in the acquired hardness level was linked to the micro-hardness alterations that occurred during all heat treatment regimens.
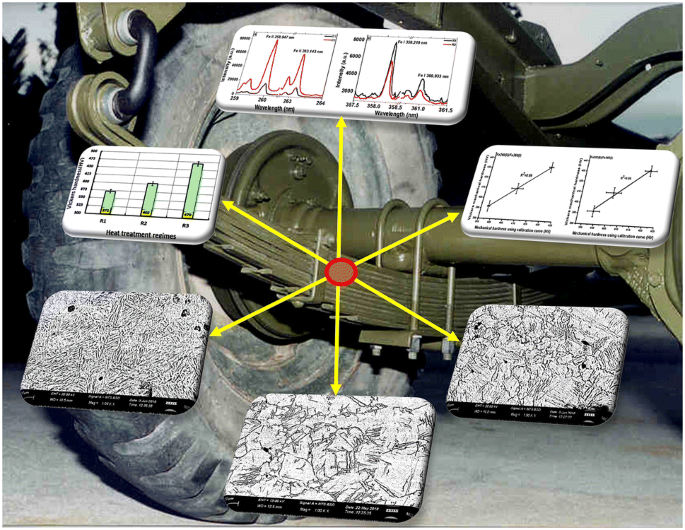
When performing qualitative and quantitative atomic emission analyses of various objects, it has been realized that single and double-pulses LIBS is the most reliable technology due to its fast-developing nature. Voropay and Ermalitskaia argue that the technique has benefits of laser-induced substance evaporation and ablative plasma excitation (1). Due to these benefits, the method has increasingly been used to determine the elemental concentration in industrial samples, natural items, building materials, cultural treasures, and works of art. However, LIBS depends on the features of the model, the laser’s settings, and the unique characteristics of multicomponent ablative plasma propagation, mainly when double laser pulses are used. Additionally, Voropay and Ermalitskaia demonstrate that ablation does not depend on the physical and chemical characteristics of the sample at flux levels typically utilized for analytical purposes. However, the plasma-second pulse interaction parameters may cause the propagating ablative plume to exhibit geographical and temporal heterogeneities (Voropay and Ermalitskaia 2). Therefore, regional heterogeneity of multicomponent ablative plasma could lead to sizeable quantitative analysis errors, particularly when utilizing a calibration-free method based on the derivation of the Boltzmann expression for the line emissivity.
Numerous studies have revealed that after double-pulse ablation of brass and bronze, analysis of the elemental distribution on the adsorbed plate does not have a homogenous deposited layer. Voropay and Ermalitskaia claim that there are differences in concentration of elements in deposition compared to the components in the original sample (4). Further on, their study revealed that the laser beam’s ablative plume extends down its axis. At the same time, low-melting elements like zinc, lead, and tin expand primarily in a small central plasma zone that passes through the adsorber plate’s hole without deposition (Figure 6). This indicates that low-melting component velocity is higher along the laser beam than perpendicular to it.
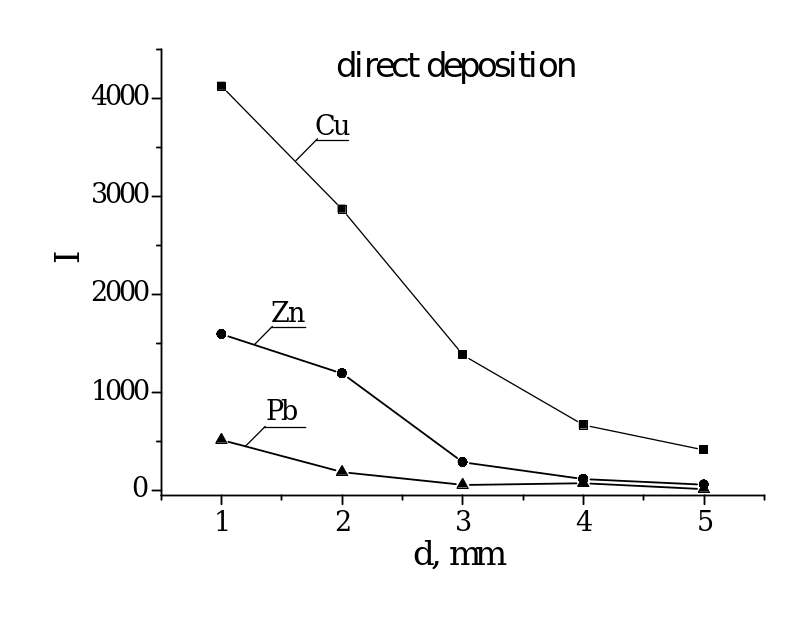
AES has been the preferred analytical method in many circumstances to ensure the geographical provenance of items. In AES, the aerosol can be directly added to the flame using a laminar burner or go through a solvent-removal stage in a separate chamber before being sprayed into the flame (Li et al. 32). The influence of tiny temperature variations on the number of excited atoms and, as a result, on the creation of free atoms is the fundamental disadvantage of utilizing a flame as an excitation source. The occurrence of spectral interferences due to overlapping spectral lines is another disadvantage.
Works Cited
Botto, Asia et al. “Applications of Laser-Induced Breakdown Spectroscopy in Cultural Heritage and Archaeology: A Critical Review”. Journal of Analytical Atomic Spectrometry, vol 34, no. 1, 2019, pp. 81-103. Royal Society of Chemistry (RSC), Web.
Dubey, Sonali et al. “Rapid Analysis of Chemical Composition and Physical Properties of Gemstones Using LIBS and Chemometric Technique”. Applied Sciences, vol 11, no. 13, 2021, p. 6156. MDPI AG, Web.
Ermalitskaia, K, and E Voropay. Double Pulse LIBS of Titanium-Based PVD-Coatings with Submicron Resolution. Belarusian State University, pp. 1-12.
Fabre, Cécile. “Advances in Laser-Induced Breakdown Spectroscopy Analysis for Geology: A Critical Review”. Spectrochimica Acta Part B: Atomic Spectroscopy, vol 166, 2020, p. 105799. Elsevier BV, Web.
Fontana, Fernando F. et al. “Integrated Laser-Induced Breakdown Spectroscopy (LIBS) and Multivariate Wavelet Tessellation: A New, Rapid Approach for Lithogeochemical Analysis and Interpretation”. Minerals, vol 11, no. 3, 2021, p. 312. MDPI AG, Web.
Förster, Daniel J. et al. “Review on Experimental and Theoretical Investigations of Ultra-Short Pulsed Laser Ablation of Metals with Burst Pulses”. Materials, vol 14, no. 12, 2021, p. 3331. MDPI AG, Web.
Lee, Chiwon et al. “Disentangling Surface Atomic Motions from Surface Field Effects in Ultrafast Low-Energy Electron Diffraction”. Communications Materials, vol 3, no. 1, 2022. Springer Science and Business Media LLC, Web.
Li, Yingchao et al. “A Review of Laser-Induced Breakdown Spectroscopy Signal Enhancement”. Applied Spectroscopy Reviews, vol 53, no. 1, 2018, pp. 1-35. Informa UK Limited, Web.
Nguendon Kenhagho et al. “Characterization of Ablated Bone and Muscle for Long-Pulsed Laser Ablation in Dry and Wet Conditions”. Materials, vol 12, no. 8, 2019, p. 1338. MDPI AG, Web.
Ren How, Soo et al. “Plasma Diagnostic by Optical Emission Spectroscopy on Reactive Magnetron Sputtering Plasma –A Brief Introduction”. Journal of Physics: Conference Series, vol 1027, 2018, p. 012005. IOP Publishing, Web.
Voropay, Y.S., and K.F. Ermalitskaia. Spatial Heterogeneity of Double Pulse Laser Induced Plasma of Copper Alloys. Belarusian State University, pp. 1-10.
Zhang, Quanli et al. “Surface Micro-Texture on Sapphire Fabricated by Laser Ablation Trajectory Regulation”. Chinese Journal of Aeronautics, vol 35, no. 3, 2022, pp. 525-536. Elsevier BV, Web.