Background
Spectroscopy is an example of a forensic technique used to detect, in many cases, determine the composition and the quantity of a certain compound in a sample(Siegel 1997). Spectroscopy is defined as the measurement of the absorption, emission, or scattering of electromagnetic radiation from a surface by atoms or molecules (Siegel 2004, p. 2326). Absorption is the transmission of electromagnetic energy to the atoms or molecules; emission is the transition of electromagnetic energy from one energy level to another resulting in photon emission and scattering is when light is redirected when it interacts with matter (Skoog, Holler, & Nieman 1998).
Principle
When a primary X-ray beam of precisely known energy impinges on sample atoms, inner shell electrons are ejected and the energy of the ejected electrons is measured. The difference in the energy of the impinging X-ray and the ejected electrons gives the binding energy (Eb) of the electron to the atom. Since, this binding energy of the emitted electron depends on the energy of the electronic orbit and the element it can be used to identify the element involved. Further, the chemical form or environment of the atom affects the binding energy to a considerable extent to give rise to some chemical shift, which can be used to identify the valence state of the atom and its exact chemical form. This technique is mostly referred to as Electron Spectroscopy for Chemical Analysis (ESCA). ESCA is a well-established surface spectroscopy technique to provide effective chemical analysis of surfaces in given specimens. The method is of valuable use when elements of low atomic number and surface impurities are considered.
The method is complicated and requires relatively high vacuum (UHV) conditions. The UHV conditions are typically below 10 to the -9 millibar. The atmospheric pressure is just 1 bar and therefore at UHV conditions the number of atoms is 1/1,000,000,000,000 that of air per unit volume. This condition is highly recommended for surface analysis as the molecules in the air will land to the surface and change the surface property. The atoms are attached to the surface in 3 seconds and cannot be analyzed since this time is not enough to experiment. The UHV conditions are required to provide several hours for the experiment to be completed. The UHV chamber is first prepared by pumping 10 to the -2 millibar using a rotary pump. A turbomolecular pump is then used to pump 10 to the -6 millibar. The vacuum chamber is enclosed in a tight oven and heated to about 180 degrees Celsius. The baking takes place for two days to remove any gas inside the chamber and it is then allowed to cool down. This attains the UHV chamber at room temperature. The output (results) is analyzed by identifying characteristics peaks as well as analyzing the peak areas. The instruments are linked to computers to assist in data analysis. The process of adhesive encompasses the surface condition and composition. ESCA or XPS has provided forensic scientists with an important tool to recognize the causes and location of failure.
Introduction
Electron spectroscopy for chemical analysis (ESCA) also known as x-ray photoelectron spectroscopy was first explained in 1905 by Albert Einstein (Wagner, et al. 1979). Einstein demonstrated that the energy of an electron ejected in the photoemission is equivalent to the difference between the energy of the target photon and its binding energy. The rapid development of ESCA was actualized when researchers measured the binding energies of the core electrons by x-ray photoemission and realized that these binding energies could vary as much as 6 eV depending on the chemical state of the atom. This technique provides important information about the chemical effects on surfaces making it an extremely surface-sensitive technique (Sibilia 1988). Other spectroscopy techniques such as Atomic emission spectroscopy (AES), Atomic absorption spectroscopy (AAS) among others interpret absorption or emission as a function of energy whereas ESCA measures the kinetic energy of the electrons which are ejected by x-ray radiation (Seah & Briggs 1992).
The ESCA is performed using a synchrotron-based light source that is combined with a custom-designed electron analyzer (Siegel 2000). The most highly used ESCA system consists of 20-200 micrometer beams of monochromatic (aluminium K-alpha x-rays) or polychromatic (magnesium alpha x-rays). The monochromatic beam of x-rays is produced by diffracting and focusing a polychromatic beam of x-rays using a thin disc of naturally occurring crystalline quartz with a <1010> lattice. The resulting wavelength of 0.8339 nm corresponds with the photon of 1486.7 eV. These monochromatic x-rays have a diameter of 0.16 eV but the ESCA system has a high energy resolution of about 0.25 eV. On the other hand, the polychromatic magnesium x-rays produce a wavelength of 0.99 nm which corresponds to the photon energy of 1254 eV. This non-monochromatic x-ray is approximated as 0.70 eV which is the energy resolution of the system when using this type of a beam. In addition, crystals are not used to diffract the x-rays and this allows all the primary x-rays and high-energy Bremsstrahlung x-rays to reach the surface (Ewing 1985).
ESCA is widely used in forensic laboratories. It is mainly used for surface analysis, especially in the qualitative identification of the elements in a sample. Based on the chemical shifts, the chemical environment around the atoms can also be estimated. This measurement is useful in determining the valence states of the atoms present in various moieties in a sample. Quantitative measurements can be made by determining the intensity of the ESCA lines of each element.
Instrumentation
It consists of a radiation source for primary X-rays, monochromator, an energy analyzer (to resolve the electrons generated from the samples by energy) and a detector to measure the intensity of the resolved electrons. The analysis is done in high vacuum (Kuwana 1980; Ingle & Crouch 1988). Figure 1 below shows a schematic diagram of the ESCA system.
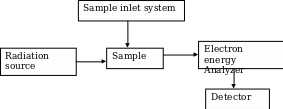
The ESCA system is routinely used to analyze a wide variety of compounds such as inorganic, polymers, elements, make-up, teeth, bones and many others (Schroder, Muller & Arndt 1989). The surface composition, usually 1 to 10 nm, is analyzed to find its empirical formula, elements that contaminate the surface; line profiling that analyzes the uniformity of elemental composition across the top surface as well as the depth profiling (Levy & Lemeshow 1991). The photoelectrons emitted from the sample are counted at each kinetic energy value and a spectrum is generated from equation 1. Since the binding energy of an electron is its chemical characteristic of the specific element, orbital, ESCA is also used in determining the bonding state and the surface concentrations.
Methods
The (XPS) also known as ESCA uses the concept of photoelectric effect to detect the chemical composition of a surface. Some special ESCA systems have been designed to analyze volatile gases or liquids, samples at different temperatures and at approximately 1 torr vacuum. The fact that the energy of a particular x-ray wavelength is known, the electron binding energy (BE) of each emitted electron can be determined using the following equation that is based on the work of Rurherford;
Ebinding = Ephoton – Ekinetic – φ (1) where Ebinding is the energy of the electron emitted from one energy level to another within an atom, Ephoton is the energy of the photon used, Ekinetic is the kinetic energy of the emitted electron measured by the electron and φ is the work function of the instrument. The purpose of a work function is to provide extra energy used to transfer the electron from the specimen surface to the vacuum area. Each instrument has its own predetermined value.
Fundamentally, there are three stages or activities involved in the analysis of sample. Firstly, the sample is collected from the source. This is followed by preparation of the sample which may include techniques like culturing, cleaning or soaking and thirdly using appropriate methods for analysis. These techniques are independent of each other but one can have an effect on the other. In analysis, the error is ostensibly possible and the person analyzing should be alert to identify these errors and avoid them. The method of analysis to be used should address the level of accuracy, sensitivity and dependability. The scientists should therefore evaluate the method at hand and decide whether it is acceptable or not.
Sample preparation
The solid samples to be analyzed should be 0.1-4.0 cm2 and with very low vapor pressures (Yacobi, Holt & Kazmerski 1994). The samples are first analyzed and the surface of 20Å is removed by light sputtering (known as depth profiling). This guarantees that the surface is not contaminated by any organic or incidental impurities. In forensic analysis, a quality assurance program must monitor the testing procedural and make certain that testing techniques applied and results reported pass the proficiency and auditing test. The guidelines are found in the trace evidence quality assurance guidelines (TEQAG).
Sample analysis
In ESCA, the sample is irradiated with a monochromatic beam of x-ray. The core electron(s) of the sample are emitted by the well-known concept of the photoelectric effect. The concept is explained by the fact that when a metal surface is irradiated with light, electrons are ejected. The emitted electrons are detected using a cylindrical mirror analyzer (CMA) detector. The kinetic energy given is then converted to binding energies thus allowing the identification of the present elements. The resulting energy spectra are given as binding energy versus intensity. The peak intensities may give valuable quantitative information about the elemental surface compositions if sensitivity factors are employed. ESCA or XPS is widely used in forensic laboratories to analyze adhesive joints and obtain compositional information on the specimen surfaces. This is particularly useful in the identification of contaminants that may have caused the failure, non-bonded areas, and lack of adhesive. In addition, it also checks whether the failure is on the adhesive or original material or at the interface.
Results
The ESCA spectrum provides information about the surface of the sample as well as the oxidation state (chemical environment) of the element. The strength that binds an electron is influenced by the chemical environment surrounding it. Such atom with dissimilar chemical environment generates peaks with different binding energies which are referred to as chemical shift. Moreover, separate chemical states disentangled using peak fitting programs to give the percent composition of an individual state. While reporting the laboratory results, uniformity and consistency should be observed. The format, accepted units of measurement and calculation should be clearly documented in the laboratory manuals. All the persons involved in the investigations should be able to understand the results and interpret them giving the significance very clearly. In addition, laboratory results should be ISO (International Organization for Standardization) compliant. This body requires that the reported information be concisely clear, of high accuracy and instantly recognizable in the presentation of results.
Advantages
Basically, both the ESCA technique and the Auger Electron Spectroscopy (AES) are surface analysis techniques. The CMA detector is used to analyze the ejected electron in both techniques. In addition, the ESCA scan provides similar or comparable information to AES. However, the ESCA have a number of advantages over AES and this have recently made ESCA a good complementary method to AES in forensic analysis of biomolecules. For example, the AES may give destructive electron bombardment to some materials which on the contrary are not affected by ESCA monochromatic x-ray bombardment beam. Further, the electron bombardment in AES has a problem of charging the insulative specimens which leads to poor analysis. The charging up of specimen is never experienced in ESCA neutral analysis.
The resolution energy of ESCA is typically better than that of AES. This is particularly useful in detecting of binding energies of atoms in a molecular structure. Thus information on elemental composition as well as chemical bonding is given. ESCA has widely been applied in the semiconductor industries to study the surfaces of organic, oxides and polymers. It has also been employed in development of plasma etching techniques.
Limitations
The ESCA technique is unable to detect the hydrogen atom or helium atom. In this technique, it is not possible to make the x-ray beam diameter smaller than that of electron beams. This inadequacy have rendered the spatial resolution of ESCA to be poor than that of AES. This inherent weakness has also led to innovation of new technique known as imaging XPS (iXPS). The modern spectrometers with this feature offer the capability of parallel imaging. This however is important as it obtains information on positions from dispersion characteristics of the hemi-spherical analyzer. This further generates images with a high spatial resolution. In recent development on the iXPS a magnetic objective lens has been introduced and this on the other hand has demonstrated improvement on the sensitivity criteria for a given spatial resolution enabling the use of the new technique to expand. In addition, the accuracy of quantitative analysis is limited to approximately 4 percent. High vacuum is also necessary for the system to avoid the low energy electrons to be collided with other impurities, which may result in low sensitivity. It is not possible to detect the impurities at the ppm or ppb levels (Crist 2004).
List of References
Crist, B. V. 2004, Handbooks of Monochromatic XPS Spectra, Volumes 1-5, XPS International LLC, Mountain View, CA, USA.
Electron spectroscopy for chemical analysis, 2009, Encyclopedia Britannica [Online] Web.
Ewing, G. W. 1985, Instrumental Methods of Chemical Analysis, 5th edn, McGraw-Hill, New York.
Forensic Guidelines, 2000, Scientific Working Group on Materials Analysis (SWGMAT).
Garfield, F. M. 1991, Quality Assurance Principles. Association of Official Analytical Chemists, Arlington, Virginia.
Grant, J. & Briggs, D. (eds) 2003, Surface Analysis by Auger and X-ray Photoelectron Spectroscopy, Chichester, UK.
Ingle, J. D. & Crouch, S. R. 1988, Spectrochemical Analysis, Prentice-Hall Int., New Jersey.
Kuwana, T. 1980, Physical Methods in Modern Chemical Analysis, Vol. 2, Academic Press, London.
Levy, P. S. & Lemeshow, S. 1991, Sampling of Populations. John Wiley and Sons, New York.
Moulder, F., Stickle, W., Sobol, P. & Bomben D. 1992, Handbook of X-ray Photoelectron Spectroscopy, Perkin-Elmer Corp, USA.
Schroder, E., Muller G. & Arndt, K. 1989, Polymer Characterization, Hanser Publishers, Munich.
Seah, P. & Briggs, D. (eds) 1992, Practical Surface Analysis by Auger and X- ray Photoelectron Spectroscopy, Wiley & Sons, UK.
Sibilia, J. P. 1988, Materials Characterization and Chemical Analysis, 2nd edn, VCH Publishers, New York.
Siegel, J.A. 1997, ‘Forensic Chemistry’ in Macmillian Encyclopedia of Chemistry, Macmillian Company, New York.
Siegel, J.A. 2000, Collection and Chain of Evidence, Academic Press Limited, London.
Siegel, J.A. 2004, ‘Accreditation of Undergraduate and Graduate Forensic Science Education Programs’, Forensic Magazine, Vol. 1, No. 3, pp. 2326
Skoog, D. A., Holler, J. & Nieman, T. A. 1998, Principles of Instrumental Analysis, 5th edn, Thomson Learning, Crawfordsville.
Surface Chemical Analysis — Vocabulary, ISO 18115: 2001, International Organization for Standardization (ISO), TC/201, Switzerland
Wagner,C.D., Riggs, W., Davis, L. & Moulder, G. 1979, Handbook of X-ray Photoelectron Spectroscopy, Perkin-Elmer Corp., Eden Prairie, MN, USA
Yacobi, B., Holt, D. B. & Kazmerski, L. 1994, Microanalysis of Solids, Plenum Press, New York.