Introduction
Biomedical engineering has of late been widely acknowledged and applied across the major facets of life like in industries and medicine research; these applications have been majorly appreciated in the medical fraternity. Improved diagnosis of some diseases which could not have been effectively achieved is on the verge of attaining full support from the application of diagnostic techniques like wide field confocal microscopy, full field optical coherent tomography and ultrasound using 2-D phased-array transducer. These methods share amongst other many features the ability to improve image resolution by ensuring the right speed is put under utilization. It is projected that sooner or later these methods are going to replace the conventional methods that are currently in use. However these novel technological advances also comes with major disadvantages that limits their large scale application, for instance they are all very expensive, they require a skillful personnel to operate which would require additional specialized training, they are also complex and hard to maintain, this paper will look at the basic principles applied by each of these techniques to achieve their functions, compare their advantages and disadvantages and finally look at the possible areas where the improved speed is applied in real life.
Wide Field Confocal Microscopy Using Structured Illumination Method
Amongst other important features, this microscope can utilize a high speed tandem Scanning reflected light microscopy where a stabilized configured objective is employed to attain a required high speed (Watson 1994, p. 168). A substitute for this in achieve the high speed requirement involves the use of a video rate laser scanner which would have an added advantage of being able to control the size of the aperture and the laser intensity.
This improved speed coupled with the ability to eliminate any background information from the plane of focus and therefore leading to reduced image degradation is a key feature that improves the quality of image (Langhorst et al. 2009, p. 858; Gustafsson et al. 2008, p. 4960). There are other features like serial collection of specimens that are optic tied to this technique. When using this microscopy, it is possible to quantify the depth for it adds a third dimension when it images only one plane within the sample at a time (Liang et al. 1997, p. 751).
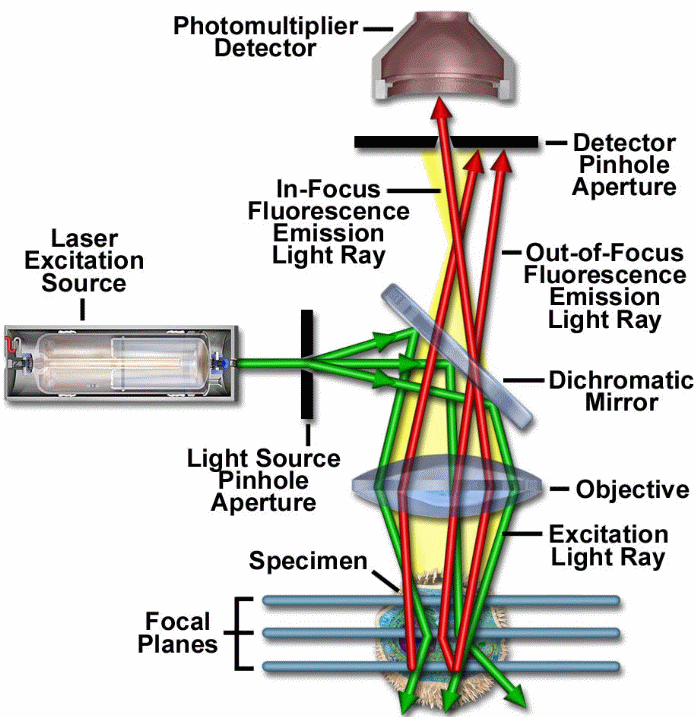
From the above schematic representation, one can deduce the main principle and how the technology achieves its function, the laser system or the excitation source emits coherent light which is directed to pass via an aperture. The aperture would be found situated on a conjugate plane, detector (a photomultiplier tube) front will act as the second position of the pin hole aperture for scanning (Toomre and Pawley 2006, p. 222).
This laser would be reflected from a dichromatic mirror, for scanning the biological sample under test, and this would be found aligned in a certain focal plane. In this same focal plane on the sample, the secondary fluorescence would be emitted and pass via a dichromatic mirror, these would then be focused on the detector aperture as confocal points (Liang et al. 1997, p. 751).
Advantages
A key advantage is that the technology utilizes optical sectioning; this would help in removing or getting rid of the artifacts which usually occurs when physically sectioned or when the specimen to be processed is also physically stained with fluorescent stains as in the case of traditional microscopy. Not only is the clarity enhanced in this non-invasive optical technology, but it also useful when examining either a living or a fixed specimen over a wide field of conditions (Gustafsson et al. 2008, p. 4962).
Disadvantages
When using a high speed setting, capturing and data recording becomes very difficult unless the data is transferred to a video microscope, analogue videos obtained are hard to handle when digitalizing before processing as well (Watson 1994, p. 172).
Resolution is limited by the component numerical aperture, both incident and detected light wavelengths (Toomre and Pawley 2006, p. 222). It is genuine to state that high speed images obtained cannot be represented effectively either in paper nor photographs (Watson 1994, p. 172).
Applications
The main feature is that, high speed imaging achieved by using TSM has been applied in ophthalmic imaging where speed is of essence since the retina and cornea keeps moving in vivo. Use of high speed stabilized configured objective has been applied in dentistry where real-time teeth examination is required to observe not only the remaining cracks, dentine fluid flow and cutting of hard tissues but also the interfacial regions of adhesive restoration this has helped in comprehending how these interfaces responds to stress factors (Watson 1994, p. 171; Auran et al. 1994, p. 184). It has also enabled reconstruction of the slices gotten to give a three dimensional (3D) view which would enable easy analysis of the sample volume (Hanley and Jovin 2001, p. 1115). Another importance is that any one slice is crisper and clearer than a full field fluorescence image (Toomre and Pawley 2006, p. 221). he speed factor has also been applied in ophthalmology to detect changes in optical features of human eye, speed is crucial here because human eye cannot remain static in vivo hence the time factor of examination must be very short
Full Field Optical Coherent Tomography (OCT) Imaging
This is also referred to as parallel optical coherent tomography (OCT). OCT is one of the advances in technology employed to give very high image resolutions when used to take a cross-sectional tomography imaging of inter tissues from biological samples. The devise employs the usage of an image sensor camera referred to as charged coupled device or simply CCD, which acquires the image, though it is also possible to use complementary metal oxide semiconductor sensors. The 2D target sample to be examined is usually full-field illuminated, then these lights are simultaneously collected from all the pixels, after which it is imaged with the camera to produce an image in what is known as en face orientation (Bouma and Tearney 2002, p. 6983). This orientation would be orthogonal with the optical axis and this occurs without light beam scanning. This application is believed to eliminate the possibilities of occurrence of electromechanical lateral scans (Bordenave et al. 2002, p. 2060). It is possible as well to obtain a reconstructed three dimensional representation by simply stepping the reference mirror and recording the occurrence of successive en face images. The image size is not a serious problem to full field OCT because it has a parallel processing property (Shoude et al. 2008, p. 10).
This technology has been widely applied in medical imaging settings; it has been used where high resolution images are desired to achieve maximum therapeutic approach (Fercher 1996, p. 158). Major it has been applied in ophthalmology to visualize highly resolved retina images and other segments of the eye (Hoerauf and Bimgruber 2002, p. 487). It has also been used in industries where non destructive testing is desired.
Principle of operation
The principle applied is based on the interference occurring between the light originating from the source to the test object with the light reflected back by this object through a beam splitter or a semi-transmissive mirror. This depends on phase shifting method used in extracting interference fringe envelop within the white light microscopy (Bouma and Teary 2002, p. 67). The fringe envelop would be obtained when several interferometric images are combined once the introduction of discrete or continuous temporal phase shift. Phase stepping method would involve using a known stepping amount when measuring the intensity. The inertia force would limit the operation speed if mechanical displacement was used to induce the phase shift (Bouma and Teary 2002, p. 69).
Its long measurement time limits the possible application of this machine in studying the static objects. The system employs the use of integrating bucket technique; this involves the integration of intensity as the phase is shifted continuously. Shifting would occur in a linear saw-tooth like method, this would enable recording of several buckets or integrated values of intensity. Integrating technique is better than phase stepping because it allows faster operation. An increase in speed would be realized in this case.
Some researchers have come up with findings to improve the speed upon which this machine can be applied, for instance Dubois and Bocarra (2009, p. 23) developed a method that could extract the fringe envelop more rapidly compared to those found in conventional methods. This is because increased speed is paramount when taking measurements from biological materials that do not remain static over longer periods of measurement. They used a sinusoidal phase modulation method where the reference mirror was made to oscillate together with four integrating buckets (Dubois and Boccarra 2009, p. 23).
Application
This method can be applied in industries where quality assurance and quality control are desired since the method gives cross sectional images from the biological materials or any other samples under test without damaging or touching it. It can also be applied in monitoring continuous productions and assembly in a manufacturing plant.
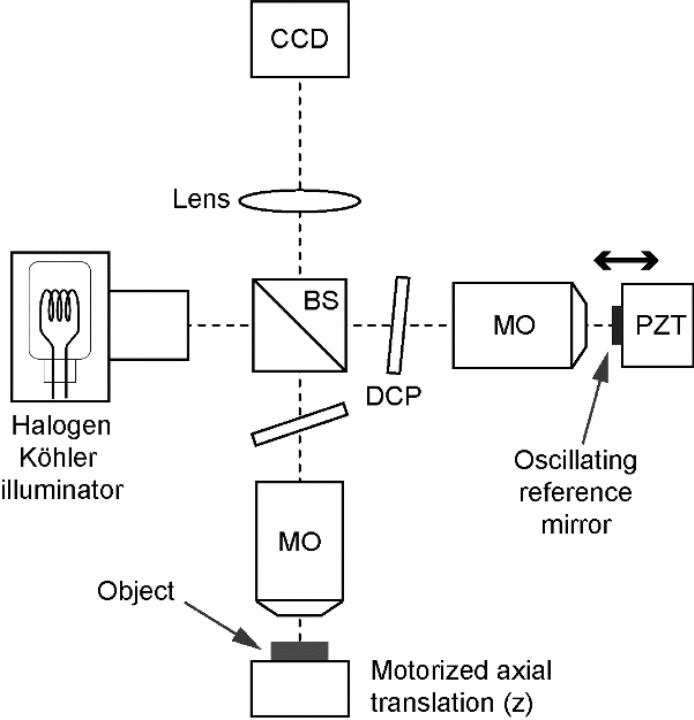
The equation below summarizes the axial resolution which depends on the coherent length of the illumination process; this is inversely proportional to the spectral bandwidth;
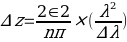
Where represents the media refractive index,
represents the centre wavelength and
represents the spectral width (Dubois et al. 2004, p. 2876).
Ultrasound Using 2-D Phased-Array Transducer
The rate at which data is acquired by this apparatus will depend on the receive mode parallel processing in both steering dimensions of Ө and Ф. Alternatively, addition of ratio delay lines to the principle electronic will eventually add the steering angle of the receive mode (von Ramm and Stephen 1990, p. 262) and increase the rate of data acquisition. These elements are usually arranged in a certain pattern, for instance in a linear array (Turnbull and Foste 1992, p. 344). These elements can be pulsed either singly or together so as to realize a pattern of wavefront; these wavefront will interfere before eventually giving rise to a common beam profile (Saleh and Smith 2004, p. 9). This profile could be varied by varying wave height as well as the duration each element takes to be excited within the beam profile. One major attribute is that there is a software control of the amplitude; this is coupled with a time delay for each of the elements, in achieving this, focal law will play a major role (Ditchburn and Ibrahim 2009, p. 56).
This technique has been applied in medical fields where a non destructive evaluation is desired, and chances are that it is the technology on watch with most expectation of replacing other conventional methods that are currently employed in medical diagnostics (Bulavinov et al. 2010, p. 68).
Time resolution would play a significant role in resolving small structures of the biological material. Since a highly resolved visualization of side to side targeted material is required, then well focusing will aid in achieving this. But most importantly, when the pulse has a shorter length then resolution of tiny targets separated with shorter distances between is possible in any biological sample (Fenster et al. 2001, p. R69).
According to Ditchburn and Ibrahim (2009, p. 57) beam profile modification produced by phased array probe achieves three major techniques that have been used in scanning these are underlined below.
- Linear scanning, this involves a division or a cluster of the ‘array elements’ which are usually pulsed so as to form a profile of beams, once this is in place the focal law producing this profile would be multiplexed electronically along the entire array distance.
- Dynamic depth focusing, this involves variation of the focal law by moving the focal points electronically along the beam axis.
- Swept angular (sectorial or azimuthal) scanning, in this case the focal laws would be chosen, this would be made to bring about beam steering on a fixed angle with the coming ray or could be made to just sweep the beam over a wider angular range.
These advanced scans have been modified and programmed so as to relatively suit the inspection with e ease. The operator would be required to specify the expected distance of the intended beam focal as well as patterns of scanning, though elements’ time delays would be given by a machine’s software employed (Sharifi and Zadeh 2004, p. 21).
Advantages
High speed utilization which ensures increased rate of data acquisition has been applied in 3D imaging where a 2D image would be reconstructed to give a volumetric image. This feature has made it possible to size tumors, locate masses and have a realistic visualization of various biological parts under examination in medical diagnosis (von Ramm and Stephen 1990, p. 264). Increased sensitivity and coverage are realized by its ability to control the shapes and directions of the beam generated by the machine. Since the complex scan allows for interrogation of a larger capacity of material within a probe, then increased coverage will be realized (Ditchburn and Ibrahim 2009, p. 57).
Their ability of producing immediate images would not only allow a straightforward visualization on the biological internal structure but also simplify interpretation the gotten data. Although this feature is also found in conventional instruments that have been based on mechanical scanning systems, this particular one is mainly restricted to a larger-scale or advanced system applications (Fenster et al. 2001, p. R69; Turnbull and Foste 1992, p. 344).
Disadvantages
As expected, application of high speed would require the technician to read the data gotten very fast and comprehend the information presented, however, this is not possible with human beings. Maintaining the plane of focus is tedious because of the comprehensive interface occurring between the cornea and the contact objective (von Ramm and Stephen 1990, p. 265). This apparatus is also expensive for purchase, the probe is even worse because it costs over five times more than what a single-crystal transducer would cost. It requires skilled personnel to operate it and correctly interpret the acquired wide range of data presentation gotten from the system, which may be very difficult to most users. In case where an inexperienced operator is tasked with its usage, then chances are that there might be limited efficiency of the machine due to inappropriate selection of its settings. Equally, the operators may have a misconception and conclude that a scan represented by a sector which normally arises from a probe stationed stationary would be able to test all the discontinuities within the sweep range.
Another demerit would be attributed to their lack of an accepted inspection criteria and standards as well as calibration blocks which can be applied to these phased arrays. When setting up the apparatus for the first time, one would really take time in getting everything right. Focal law settings like determination of inspection angle, scan patterns as well as focal distance amongst other parameters would really require a keen and thorough review (Bulavinov et al. 2010, p. 69). But once these have been done it is usually possible to save the program for subsequent retrieval in future inspection if need be.
Their large probe dimension also poses some disadvantages in that one would find it hard to attain a good ultrasonic coupling from the inspection surfaces. For this to be achieved therefore, waviness and surface condition of the biological material to be inspected becomes an important factor when using a phased array (Bulavinov et al. 2010, p. 69). When the beam is focused too shallowly, chances are that a deeper discontinuity would be missed.
Application
The key features enabling the application of this apparatus is high numerical aperture brought about by its high light gathering capabilities as well as optical scanning and resolution. These features are attributed to high speed application in examination of biological tissues and cells that are always in constant motion, speed would therefore be of importance when in vivo testing is to be carried out, such medical specialties are; examination of cardiac functioning, examination of cornea and retina (von Ramm and Stephen 1990, p. 265).
Conclusions
If these novel technological advances are fully integrated into their various applications, then life would improve for all the people likely to use it. It is however important for key research on both their known and unknown areas as of applications to be inducted and made clear, these would not only make them safe for human consumption but also fit in the ethics of science. Biomedical science and engineering has proved to be.
Reference List
Auran, D. J., Koester, J. C., Raparport, R & Florakir, J. G.,1994. Wide field scanning slit in vivo confocal microscopy of flattening-Induced corneal bands and ridges. Scanning 16(3), pp. 182-186.
Bordenave, E., Abraham, E., Jonusauskas, G., Tsurumachi, N., Oberle´, J., Rulliere, C., Minot, P.E., Lassegues, M. and Surleve B.J.E., 2002. Wide-field optical coherence tomography: imaging of biological tissues. Appl. Opt., 41, pp. 2059– 2064.
Bouma, B.E. and Tearney, G.J., 2002. Optical source. In: E. Bouma and J. Tearney, eds. 2002. Handbook of Optical Coherence Tomography. New York: Marcel Dekker, pp. 67–97.
Bulavinov, A., Pinchuk, R., Pudovikov, S., Reddy, K.M. and Walte, F., 2010. Industrial Application of Real-Time 3D Imaging by Sampling Phased Array. Moscow: European Conference for Non-destructive Testing.
Ditchburn, R.J. and Ibrahim, M.E., 2009. Ultrasonic phased Array for inspection of Thick- sectioned welds. Victoria-Australia: Maritime Platforms division.
Dubois, A. and Boccara, A.C., 2009. Full-field Optical Coherence Tomography. Applied optics, 63(11), pp. 48-60.
Dubois, A., Greeve, K., Mooney, G., Lecaque, R., Vabre, L. and Boccara, C., 2004. Ultahigh resolution full-field Optical Coherence Tomography. Applied optics, 43(14), pp. 2874-2883.
Fenster, A., D´onal, B.D. and Neale, H.C., 2001. Three-dimensional ultrasound imaging. Phys. Med. Biol., 46, pp. R67–R99.
Fercher, A.F., 1996. Optical coherence tomography. J. Biomed, Opt., 1, pp.157–173.
Gustafsson, M.G.L. et al., 2008. Three-Dimensional Resolution Doubling in Wide-Field Fluorescence Microscopy by Structured Illumination. Biophysical Journal, 94(12), pp. 4957-4970.
Hanley, Q.S and Jovin, T.M., 2001. Highly multiplexed optically sectioned spectroscopic imaging in a programmable array microscope. Applied Spectroscopy, 55, p.1115.
Hoerauf, H. and Birngruber, R., 2002. Optical coherence tomography in the anterior segment of the eye. In: E. Bouma and J. Tearney, eds. 2002. Handbook of Optical Coherence Tomography. New York: Marcel Dekker, pp. 487–503.
Langhorst, M., Schaffer, J. and Goetze, B., 2009. Structure brings clarity: Structured illumination microscopy in cell biology. Biotechnology Journal, 4(6), pp. 858-865.
Liang, M., Stehr, R.L., and Krause, A.W., 1997. Confocal pattern period in multiple Aperture confocal imaging systems with coherent illumination. Optics Letters, 22, pp. 751-753.
Saleh, K.Y. and Smith, N.B., 2004. Two-dimensional ultrasound phased array design for tissue ablation for treatment of benign prostatic hyperplasia. Int. J. Hyperthermia, 20(1), pp. 7–31.
Sharifi, H. and Zadeh, S.H., 2006. New 2D ultrasoumd phased array design for hyperthermia cancer therapy. Int. J. Hyperthermia, 12(3), pp. 18-26.
Shoude, C., Sherif S., Mao, Y. and Flueraru, C., 2008. The Large Area Full-Field Optical Coherence Tomography and its Applications. Open Optics Journal, 2, pp. 10-20.
Toomre, D. and Pawley J.B., 2006. Disk-scanning confocal microscopy. In: J. Pawley, ed. 2006. Handbook of Biological Confocal Microscopy. New York: Springer Science+Business Media, LLC, pp. 221-238.
Turnbull, D.H. and Foste, F.S., 1992. Simulation of B-Scan Images From Two Dimensional Transducer Arrays: Part Ii-Comparisons between Linear and Two- Dimensional Phased Arrays. Ultrasonic Imaging, 14, pp. 344-353.
von Ramm, T.O. and Stephen, S.W., 1990. Real time Volumetric Ultrasound Imaging System. Journal of Digital Imaging, 3(4), pp. 261-266.
Watson, T.F., 1994. Application of high speed Confocal Imaging Technique in Operative Dentistry. Scanning, 16(3), pp. 168-173.