Abstract
Laser communication is the fastest form of data transfer, known to humankind. A laser beam transmits electrons in a narrow path in a straight line, which can then be caught by a receiver and interpreted as data. Low-frequency lasers are commonly used in high-speed internet cables in order to avoid various shortcomings of an unprotected laser beam. Lasers are also used in ground-to-satellite and satellite-to-satellite communications.
However, numerous issues and complications make laser communications in space less feasible. Some of them include the accuracy of the impulse, energy requirements, and data losses along the way. Mathematical formulas and explanations are given to explain each of these phenomena and provide a framework for understanding and calculating their impact in a space communications project.
Introduction
Light is the fastest form of energy known to humankind. Because of the speed which greatly surpasses that of sound as well as electric and magnetic impulses, it is frequently used in communication. On the ground, due to the Earth’s curvature and a multitude of solid and gaseous obstacles, beam communication is used only for short distances.2 Long-distance communication is done via optic fibers, which are wires made out of strands of glass that help conduct light and use it to transmit data and information. Although the light’s speed is severely reduced due to a multitude of refractions from the walls of the glass fibers, it remains the fastest mode of communication available.14
However, when it comes to space, matters are different. There are numerous satellites orbiting our planet. Communication with them is done primarily via radio signals, as those can ignore the clouds and other visual obstacles that may greatly reduce the effectiveness of lasers launched from the ground.9 Nevertheless, ground-to-space laser communication shows much promise as a faster and more accurate way of controlling satellites. Inter-satellite laser communication is also a great prospect to explore, as there are no obstacles in space that could impede or reduce the effectiveness of a laser beam. The purpose of this paper is to reflect on the use of lasers in communication and provide mathematical data on the mechanisms behind their use.
What is a Laser?
LASER, or lightly amplified by stimulated emission of radiation, is a concentrated beam of electrons launched in a single direction.5 It is different from normal light in that it is concentrated and has a singular wavelength, meaning that some lasers are invisible to the human eye. Lasers are usually generated using special glasses, crystals, or gas lamps, which contain atoms with electrons, which are then ripped off and funneled into a single beam to create a laser.
This is done by processing an electric current through the electron-generating material or substance. As a result, the beam is capable of transmitting much higher concentrations of energy and power when compared to a dispersed beam of light. It enables lasers to be used in a variety of fields, from communication to ophthalmology.
The Basics of Laser Communication
Lasers start forming concentrated beams of electrons only when the electricity running through the electron generator is higher than a certain threshold.12 From there, the optical power of the laser grows, the higher the current is. The relationship between power output and current is represented in Figure 1:
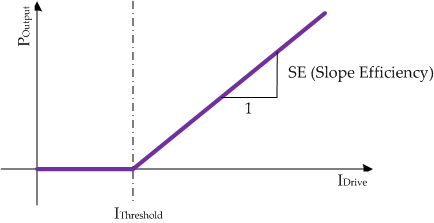
As it is possible to see, until the threshold is exceeded, the laser produces no optical power. When the electric current exceeds the threshold, the optical power produced by the laser is proportional to the level that ITr is exceeded by the current. The proportional coefficient that represents the relationship between PO and ID is called Slope Efficiency (SE).10
Power Output and Temperature
One of the main reasons why lasers are much more useful in space than in the atmosphere lies in the relationship between power and temperature. The more output the laser has, the more reliably it could transfer energy to the receiver. The power is calculated using the following formula:4
- PO = SE*(ID – ITr), if ID > ITr, otherwise PO = 0
However, ITr is heavily interconnected with temperature. Materials have better conductivity in cold environments and less in hot environments because the chaotic movements of electrons interfere with the electrical flow. Naturally, in space, the temperature is much lower than on Earth, making laser emitters more efficient. The relationship between temperature and laser efficiency is presented in Figure 2:
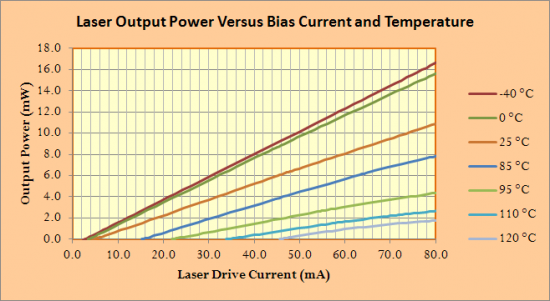
As it is possible to see, the power output at 80 mA for a laser in space is over 16 mW, whereas the same laser would have the output of about 11 mW on Earth based on temperature alone, excluding atmospheric interference. When that is taken into account, the actual output is dropped even further.
How is Laser Communication Conducted?
Optical laser receivers are considered unreliable due to various parameters that can interfere with the clarity and accuracy of the beam. In space, communication is done by sending light at two power levels. In a binary code, the higher temperature represents 1, whereas the lower temperature represents 0.6. The emitter rapidly shifts the power levels of the beam between the two in order to send a coded message containing data, which is then interpreted by the computer on the other end.
This type of communication requires increased output, as the receivers, as well as the electric conduits become hotter with time. On Earth, this presents a major problem, which makes laser installations rather cumbersome in order to possess high-capacity accumulators to supply a higher power yield. In space, the initial temperature is much lower, and it takes a longer time for connectors and receivers to heat up. The same principle is used in Internet cables. However, since cables require a much lower power output, it is easier to control the temperature. The graph exemplifying a typical power output change is presented in Figure 3, where P0 stands for the power output necessary to transmit a zero (0), and P1 – for output necessary to transmit a one (1):
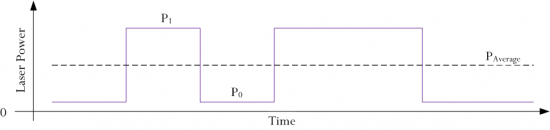
This picture, however, shows the change in power levels only over a short period of time or in the presence of temperature control mechanisms, otherwise the power output will be expected to rise with time. The new, dual-loop lasers utilize both the control of power output and temperature in order to achieve better energy use and reduce the changes in laser characteristics by vendor and lot. As a result, they are more energy-efficient.
Accuracy Issues in Satellite Communication
Accuracy issues play a significant part in ground-to-space and space-to-space communications. Normally, the power received in an by an optical dimensional link is governed by this equation:8

However, while this equation works for microwave transmitters, which have a relatively wide carrier waves and receiving antennae, it is not very applicable for lasers due to relatively small area of the beam and the receiver.13 It requires high accuracy and alignment of both the transmitter and the receiver in order to achieve maximum output. According to Leeb,8 the important parameters in this calculation include the diameter of the transmitter and receiver antennae, the full beam divergence, and point-ahead angle. These parameters are calculated using the following formulas:8
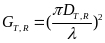
– full beam divergence
– point ahead angle
Because of the amount of variables involved, it is difficult to design an automatic system that would account for the point ahead angle in satellite – satellite and Earth-satellite communication.3 As it stands, point ahead angles are calculated using the known ephemeris data, which are used in an open loop control system. This creates additional complications and reduces the effectiveness of laser communication systems.
Data Loss Issues in Space Communications
Another issue with laser communication is that all data need to be transmitted back to Earth. There are several interferences that affect ground-to-space or planet-to-planet laser communications. These factors are vibrations, noises, and transmitting distance.11 The speed of data transfer decreases proportionately to the increase of distance between the transmitter and the receiver. This is due to light having a finite speed. If we use an example case of communication between Earth and Mars, the result would be as follows:1
R = 240 million km (distance between Mars and Earth).
DT = 0.406 m.
DR = 5.08 m.
λ=0.53*10-6m
Q*F = 0.2
F = LT * LR
α = log2 M = 5 bits per pulse
τ = 0.1 pulse width in meters
Smin = – Log 2; for PE = 10(-3), Smin = 6.2
Drate = log2 M ÷ M(∆T) = 5 ÷ 148 = 10 Mb/sec
In order to calculate PT, the data presented above would need to be put into the following formula:

PT = 1.44 watts.
This estimation was made neglecting atmospheric losses. Such calculations are necessary when estimating power banks for the laser transmitters and receivers.15 Therefore, while close-orbit laser communication is feasible, NASA considers long-range laser communication with other planets to be economically and practically dysfunctional, at our current level of technological development.7
Recommendations
The recommendations provided in this part of the paper are based on the latest advances in computing science and cybernetics. The majority of the issues presented in this paper have been known since the early 2000s. Since then, the ability to calculate point-ahead angles improved dramatically. Computers take less time to calculate all the variables before aligning the laser and the platform, in order to achieve maximum accuracy and prevent data loss.
Another matter is providing a beam with enough power to transfer data at a higher speed. As it stands, the laser beam allows for data transfers of up to 70 MBps speeds. This data transfer speed is capped by the capabilities of the drone to launch a beam of laser from planets such as Mars back to Earth. It is possible to implement a new model of lithium-ion batteries invented at the University of Illinois in 2016 to expand the drone’s laser-emitting capabilities. The new breakthrough technology enables the battery to be 2000 times as powerful compared to the ones currently in use.
The last issue is the laser’s susceptibility to atmospheric interference. Although it does not affect satellites in the planet’s orbit as much as it does potential drone missions to other planets, it would be of paramount importance in the exploration of Mars, Titan, and other planets inside and outside of the Solar System.12 Lasers require not only sources of power but also focusing lenses and optics that concentrate the beam, and which are exposed to all manners of atmospheric interference, be that extreme heat, sand, impact, or cold. The latter is especially prominent in low-temperature environments, such as Titan, which is considered to be one of the most prominent venues for space research.
Conclusions
In conclusion, laser communication in space has great potential, as it is much faster and possesses greater data transfer speeds when compared to radio waves and other forms of wireless communication. However, there are numerous issues associated with the accuracy of the impulse, energy requirements, and data losses along the way. In order to achieve maximum efficiency and make lasers feasible for long-range communications as well as to improve ground-to-satellite communications, the issues with point-ahead angles and automatic loop mechanisms must be resolved. Alternatively, optical laser receivers can be developed in order to receive laser signals such that do not rely on temperature, but rather on the change of spectrum. Therefore, prospective research in these areas is to be considered.
Works Cited
Aviv, David G. Laser Space Communications. Artech House, 2006.
Bera, Saranya, et al. “Sky to Ground LASER Looping for Communication Server Syndrome.” Emerging Trends in Electronic Devices and Computational Techniques (EDCT), 2018, pp. 1-5.
Edwards, Bernard, et al. “A Day in the Life of the Laser Communications Relay Demonstration Project.” SpaceOps Conferences, 2016, pp. 1-13.
Kaushal, Hemani et al. “Optical Communication in Space: Challenges and Mitigation Techniques.” IEEE Communications Surveys & Tutorials, vol. 19, no. 1, 2017, pp. 57-96.
Khalighi, Mohammad Ali, et al. “Survey on Free Space Optical Communication: A Communication Theory Perspective.” IEEE Communications Surveys & Tutorials, vol. 16, no. 4, 2014, pp. 2231-2258.
Khan, Fazlullah, et al. “Applications, Limitations, and Improvements in Visible Light Communication Systems.” 2015 International Conference on Connected Vehicles and Expo, 2015, pp. 259-262.
Kiasaleh, Kamran, et al. “A Novel Spatial Tracking Loop for Laser Communication Over Turbulent Unguided Optical Channels.” 2015 IEEE International Conference on Space Optical Systems and Applications (ICSOS), 2015, pp. 26-28.
Leeb, Walter R. “Laser Space Communications: Systems, Technologies, and Applications.” Semantics Scholar, 2000. Web.
Lopez-Martinez, Javier F., et al. “Physical-Layer Security in Free-Space Optical Communications.” IEEE Photonics Journal, vol. 7, no. 2, 2015, pp. 1-21.
Majumdar, Arun K. Advanced Free Space Optics (FSO): A Systems Approach. Springer, 2015.
Repe, Madhuri, et al. “Data Transmission Through Free Space Optical Laser.” Asian Journal of Convergence in Technology, vol. 1, no. 4, 2015, pp. 1-5.
Tan, Liying, et al. “Fiber-Coupling Efficiency Simulation of Gaussian Schell Model Laser in Space-To-Ground Optical Communication Link.” Optics Communications, vol. 349, 2015, pp. 112-119.
Uysal, Murat, et al., editors. Optical Wireless Communications: An Emerging Technology. Springer, 2016.
Willner, Alan E., et al. “Optical Communications Using Orbital Angular Momentum Beams.” Advances in Optics and Photonics, vol. 7, no. 1, 2015, pp. 68-94.
Zhan, Weida, et al. “Parameter Budget of DPPM and Pulse Fiber Laser in Deep Space Optical Communication.” 9th International Conference on Advanced Infocomm Technology (ICAIT), 2017, pp. 22-24.