Introduction
Magnetic resonance imaging (MRI) is a method of investigation that appeared at the end of the XX century and continues to develop intensively. It is widely used in medical and biological researches and clinical practice. In general, MRI provides an opportunity to create an image of the biological tissue, using a strong magnetic field. This technology is based on the phenomenon of Nuclear Magnetic Resonance (Bushong and Clarke 5). Based on the main principles of magnetic resonance imaging, several approaches exist to increase the speed of images obtaining, including pulse sequence, k-space-filling techniques, and parallel imaging.
The Main Principle of Magnetic Resonance Imaging
The beginning of the MRI era was connected to the discovering of nuclear magnetism. Stern and Rabi discovered this phenomenon in the 1930s and received the Nobel Prize. After that, Purcell and Bloch firstly described the phenomenon of nuclear magnetic resonance and received a Nobel Prize in 1952. Based on this principle, NMR spectrophotometers were built. In the 1960s, Damadian, using the NMR spectrophotometer in his investigation, showed the difference between spectrums of malignant and normal tissues.
In 1974, an NMR image of a rat tumor was first obtained. In 1976, an image of a human body was generated. A technology that was similar to the current one was firstly used by Lauterbur and Mansfield independently. For their discoveries, they shared a Nobel Prize. However, it was required four hours to get the first images (Bushong and Clarke 4-5). Therefore, it was essential to develop new technologies to shorter the needed time.
To create an image of the body’s tissue, a strong magnetic field is used in magnetic resonance imaging technology. Current MRI scanners produce a magnetic field which is from 1.5 to 3 Tesla while the Earth’s magnetic field is approximately 0.00005 Tesla. A scanner creates a pulse sequence – a series of changing magnetic gradient and oscillating electromagnetic fields. Produced energy is absorbed by the atomic nucleus (Constantinides 29).
In MRI, hydrogen atoms nuclei are used because they are the most common in a human body (as a part of a water molecule). After absorbing the electromagnetic energy, a nucleus emitted it later. The power of emission depends on the numbers and types of presented in the environment nuclei, in other words, on the composition of tissue (Johansen-Berg and Behrens 186).
To create an image, magnetic resonance uses the response of the hydrogen nucleus. The atom consists of a single proton and a single electron, orbiting around it. A proton has its spin which means that it rotates around its axis. If a proton is placed into a strong external magnetic field (B0), it changes its orientation and its magnetic moment (spin) aligns with the external magnetic field B0 (fig 1 a). In this position, hydrogen nuclei started to precess.
The precession of the nuclei is characterized by the frequency that is proportional to the strength of B0. This parameter is known as the Larmor frequency. It could be calculated by the Larmor equation: ω0 = γ * B0. In this equation, ω0 is a Larmor frequency in megahertz, γ0 is a gyromagnetic ratio, – constant characteristic of a nucleus, and B0 is the strength of the magnetic field in Tesla (Johansen-Berg and Behrens 12).
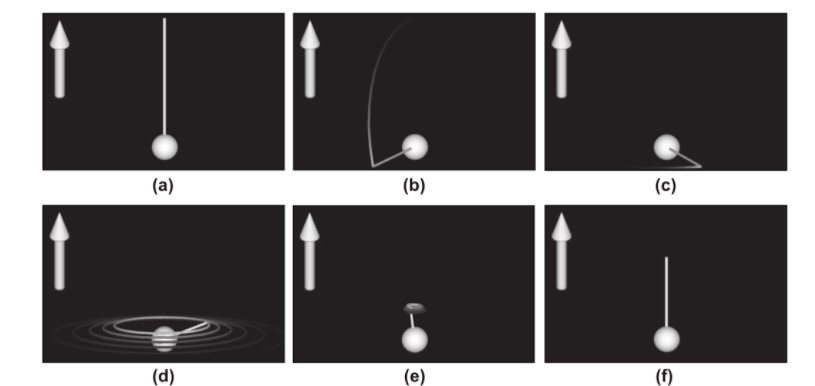
MRI scanner also generates the second magnetic field. It occurs during a short time and oscillates at radio frequencies. Therefore, it is called the RF pulse. When the RF field is turned on, the spin of hydrogen proton rotates about the axis. RF pulse induces excitation which rotates the spin away from the B0 orientation (fig. 1 b). After that, the spin precesses with the frequency according to the Larmor equation: ω=γ*B. B field is perpendicular to the B0 field (fig. 1 c). After that, precessing frequency along the B field decays. This decay lasts during the time constant T2 (fig. 2 d, e). The value of T2 depends on the type of tissue where hydrogen atoms exist. After the frequency decay, the spin returns its axis along the B0 field. It takes the time constant T1 (fig. 1 f).
Coherently rotating spins create a magnetic field and induce a current in receiver coils. This current is used to create an MR image, affecting the brightness of each pixel. The brightness of a pixel depends on the strength of a current, which, in turn, depends on the coherence of spins’ processing phases. Because the magnetic field is not completely homogenous, small differences in the frequencies of precessing spins appear, according to the Larmor equation.
After a particular time, spins start to precess incoherently. As a result, a signal loss appears. With the purpose to eliminate the incoherence of spins precessing, the second RF pulse appears at time t. After this second pulse, faster precessing spins decelerate to slower spins, and after 2t times they all precess simultaneously. The moment of spins coherence is called spin-echo (Johansen-Berg and Behrens 13). Figure 2 demonstrates how this process is reflected in the brightness of the pixel.
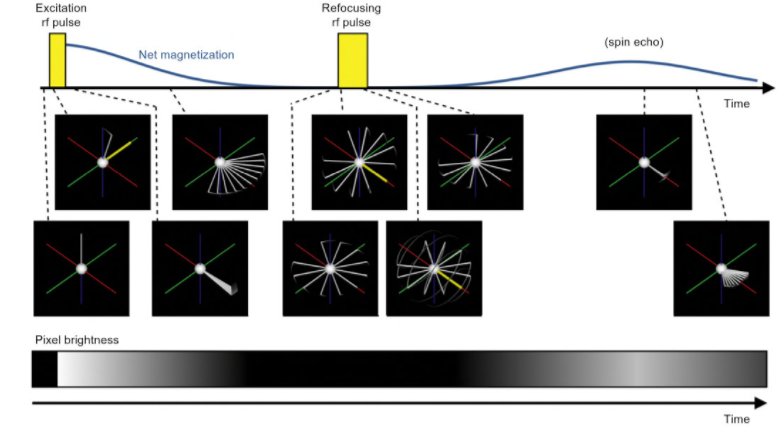
Finally, signals from precessing spins are received by the receiver coil with certain spatial encoding. Received data fill in the two-dimensional k-space. This matrix consists of Kx and Ky axes. Traditionally, during one period of time T, one Ky axes are filled in with data. The MR image is generated when the frequency data (Hz) is transformed into spatial data (mm) in the k-space. This process is called the Fourier transformation. This transformation is possible only when the whole k-space is filled (Bushong and Clarke 91).
Approaches for the Imaging Speed Increase
With the development of the MRI technique, the need for speed of imaging increased. Crucial concepts for imaging shortening are pulse sequence, k-space-filling, and parallel imaging (Moore and Chung 497).
Pulse Sequence
MRI images are obtained using the magnetic field pulsation. A certain field is turned on and of which is called “pulse sequence.” This sequence determines the quality, resolution, and contrast of the obtained images (Johansen-Berg and Behrens 11). The basic pulse sequence could be either spin echo or gradient echo. Mostly, the fast spin-echo (FSE) principle is used in clinical practice. According to this principle, after RF pulse, the series of refocusing RF pulses appear. As a result, multiple echoes are created per repetition time. The series of echoes is accelerated by the number of refocusing RF pulses (echo train length or turbo factor). As a result, instead of filling in just one line in the k-space, FSE allows filling the segment of space with several lines that correspond to echo train length (Moore and Chung 498).
The different principle is used for gradient echo. The echo is created by a single pulse with the reversal of the gradient field without the reflective pulse. “Application of a second gradient pulse of the same duration and magnitude but opposite polarity reverse this dephasing and produces an echo known as a gradient echo” (Dale et al. 70). As a result, the sequence time is shorter than for the spin-echo sequence. However, the overall signal strength will be less which is considered as a drawback of the technique (Dale et al. 71).
K-space Filling Techniques
The Fourier transformation occurs after the k-space is filled. Therefore, the rate of imaging depends on the speed of k-space-filling. Several approaches accelerate this process. In particular, single-shot echo-planar imaging (EPI) allows filling in the entire k-space in one repetition time. If multi-shot EPI is used, several repetitions times are required to fill in the k-space, which is longer. The half-Fourier imaging scheme is the scheme when only a center and top half of the k-space is filled in with the real data, and the rest is reflected from the top half which accelerates imaging.
Another possible approach for filling the k-space that is used in time-resolved MR angiography is based on the following: only the center of k-space is repeatedly filled in with the data but not the periphery. The central part of the k-space is responsible for the contrast of the image while the periphery controls the spatial resolution. Therefore, this approach allows for obtaining contrast images faster (Moore and Chung 499-501).
Parallel Imaging
For MRI, three characteristics are the most important: scan time, spatial or temporal resolution, and signal-to-noise ratio. These parameters create the “trade-off triangle” (Moore and Chung 502) that determines the quality of an image. Changing one parameter affects the other two and, therefore, the quality of a picture. However, it is possible to “broke the triangle” (Moore and Chung 502). For example, parallel imaging is a commonly used technique which allows accelerating the image acquisition without the decrease of spatial resolution. Two main methods are used: SMASH (simultaneous acquisition of spatial harmonics) and SENSE (sensitivity encoding).
A phased-array receiver coil is used for parallel imaging. Each element of the coil receives data from the entire imaging volume. It leads to redundancy of a signal which could be used to increase the speed of imaging. In theory, the maximal speed is proportional to the number of coil elements. However, it should be mentioned that the noise level is increased in parallel imaging. Therefore, this parameter limits the speed increase (Moore and Chung 503).
Conclusion
Magnetic resonance imaging is based on the nuclear magnetic resonance phenomenon. The principle of the technique is the following: in the strong external magnetic field, all protons’ spins orientate along the field line. The reflective pulse changes the orientation and spins turn perpendicular to the initial field line and start to precess with a certain frequency. These frequency data are detected and used to create the image. Several approaches are used to make this process faster: reflective pulse sequence usage, different techniques of k-space-filling, and parallel imaging. These techniques allow obtaining high-quality images in a shorter time without the resolution decrease and level of noise increase.
Works Cited
Bushong, Stewart C., and Geoffrey Clarke. Magnetic Resonance Imaging: Physical and Biological Principles. Elsevier Health Sciences, 2014.
Constantinides, Christakis. Magnetic Resonance Imaging: The Basics. CRC Press, 2014.
Dale, Brian M., et al. MRI: Basic Principles and Applications. John Wiley & Sons, 2015.
Johansen-Berg, Heidi, and Timothy EJ Behrens, editors. Diffusion MRI: From Quantitative Measurement to in vivo Neuroanatomy. 2nd ed., Academic Press, 2013.
Moore, Michael M., and Taylor Chung. “Review of Key Concepts in Magnetic Resonance Physics.” Pediatric radiology, vol. 47, no. 5, 2017, pp. 497-506.