The epoch of the internal combustion engine vehicles’ domination in the market is now giving way to the era of electric vehicles, which experience rapid growth. Such a tendency for broad-scale implementation of electric cars has significant importance for society by “moving our economies away from petroleum and lessening the environmental footprint of transportation” (Brown, 2010, p. 3797). Hence, it is crucial to study the technologies related to the subject and determine important specifications of electric vehicles.
One of the essential reasons for electric car rapid development is the global concern about greenhouse gas emissions and the associated threats of global warming. The innovative vehicles can reduce and eliminate harmful effects, contributing to the solution of the environmental problem. The purpose of this paper is to discuss the well-to-wheel efficiency of electric vehicles, the range extender, and the Vehicle to Grid technology, as well as provide a specification for a concept electric vehicle.
Part 1
First, it is crucial to consider the characteristics of an Internal Combustion (IC) engine-powered vehicle and an electric vehicle (EV). An Internal Combustion engine is a heat engine where the chemical energy of the fuel that burns in the work area is converted into mechanical work (Song and Aaldering, 2019, p. 898). Along with the electric motor, an IC engine is among the most common types of engines. As a rule, it is used in such vehicles as cars, motorcycles, trains, aircraft, and water transport. IC engines are also utilized in stand-alone electric generators to generate electricity.
An electric motor is an electric machine that converts electrical energy into mechanical. It consists of a rotating part, namely the rotor, and a fixed part, the stator (Electric vehicle basics, no date, para.2). There are electric motors of direct and alternating current, and the latter is divided into synchronous and asynchronous. Asynchronous electric motors, in turn, are divided into ones with a short-circuited rotor and a phase rotor. Furthermore, they are distinguished by function, such as general industrial electric motors, as well as crane, elevator, excavator, trolleybus, tram, and car electric motors. Furthermore, the recent accomplishments in the lithium-ion battery technology for operating range, vehicle application, and driving attitudes of BEVs are starting to meet the requirements of passenger car users.
Comparison of WTW Efficiency and CO2 Emissions between IC Engine Powered Vehicle and Electric Vehicle
Vehicles powered with different types of engines have different well-to-wheel efficiency and CO2 emissions. Well-to-Wheel (WTW) emissions are the emissions in-use due to their proportionality to the vehicle’s fuel and energy consumption (Going beyond Well-to-Wheel, 2019, para. 1). In this regard, Tank-to-Wheel (TTW) is a subrange in the vehicle’s energy chain, extending from the charging point where energy is absorbed to discharge. The difference between the two terms is that TTW refers to the use of fuel and emissions during driving. WTT indicates the subrange of fuel supply from the creation of the source of energy, such as petrol, diesel, natural gas, or electricity, to fuel supply, namely, transport to the charging point.
Well-to-Wheel is regarded as the first stage of comparing the effectiveness of various solutions in regard to greenhouse gas emissions. As depicted in Figure 1, technology transition can be observed as traditional solutions coexist with more renewable components that emerge, such as advanced biofuels and synthetic pathways. Energy consumption and fuel economy for electric vehicles have been agreed upon internally by NGVA Europe as a battery electric vehicle (BEV) consumption at 14,5 kWh/100km (Well-to-Wheel, para. 4). Overall, electric vehicles produce fewer amounts of tailpipe emissions, thus, offering fuel cost saving and lowering damage to the environment.

Different WTW efficiency is observed among vehicles based on the engine type. Figure 2 presents a comparison of WTW emissions among c-segment of vehicles, such as petrol, diesel, Plug-in Hybrid on petrol (PHEV), Battery-Electric (BEV), and compressed Natural Gas vehicles (CNG). C-segment comprises the third smallest and the so-called medium passenger cars. As can be seen, electrified solutions suggest the most efficient performance on the TTW basis, while the WTW approach indicates that BEVs offer optimal efficiency of the powertrain.
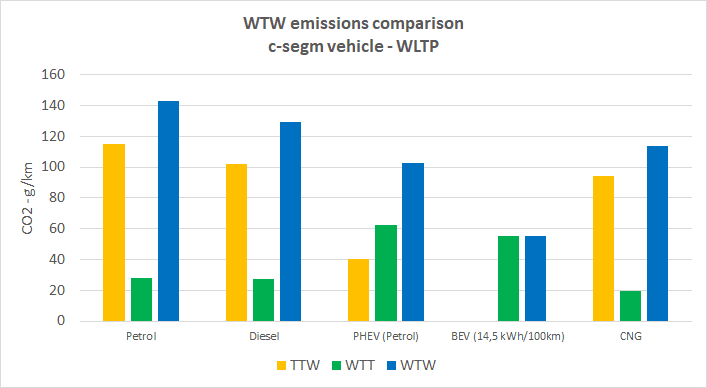
The environmental impacts of BEVs in comparison to IC engine vehicles are widely studied. EVs create considerably lower emissions than conventional vehicles across their lifetime. According to Hausfather (2019, para. 11), “around half of the emissions from battery production come from the electricity used in manufacturing and assembling the batteries.” As depicted in Figure 3, the level of CO2 emissions from these two types of engines differs by approximately 20%.
Nevertheless, ICEV-generated environmental impacts are localized to the combustion of gasoline in the engine; BEVs causes a more detrimental set of ecological effects, “offsetting a significant portion of their overall advantage with respect to greenhouse gas emissions” (Battery electric vehicles, 2016, para. 7). In this regard, IVs running on hydrogen or methane still generate slightly higher CO₂ emissions than battery-powered vehicles. But this disadvantage can turn into an advantage if renewable energy principles are implemented. Combustion engines running on fossil methane already have very low CO₂ emissions.
The structure of the energy balance of various states of the world is crucial. In this regard, “manufacturing, fuel extraction, refinement, power generation, and end-of-life phases of a vehicle, in addition to the actual operational phase” need to be considered to evaluate the greenhouse gas emission (Kawamoto et al., 2019, p. 2690). For instance, in Norway, due to the large number of natural lakes located high in the mountains, waterfalls, and rivers, almost all electricity is generated by hydroelectric power plants. Their construction does not require expensive dams, which means that it costs the authorities or private investors relatively cheap.
It is natural to build up the fleet of electric cars in such conditions because its development means a constant decrease in emissions of toxic substances from car exhaust pipes and their absence at power plants. Overall, a comparison of emissions from BEVs and IC engine vehicles are complex, but a Well-to-Wheel approach to measuring CO2 suggests that other solutions apart from electrification can also contribute to the decarbonization process.
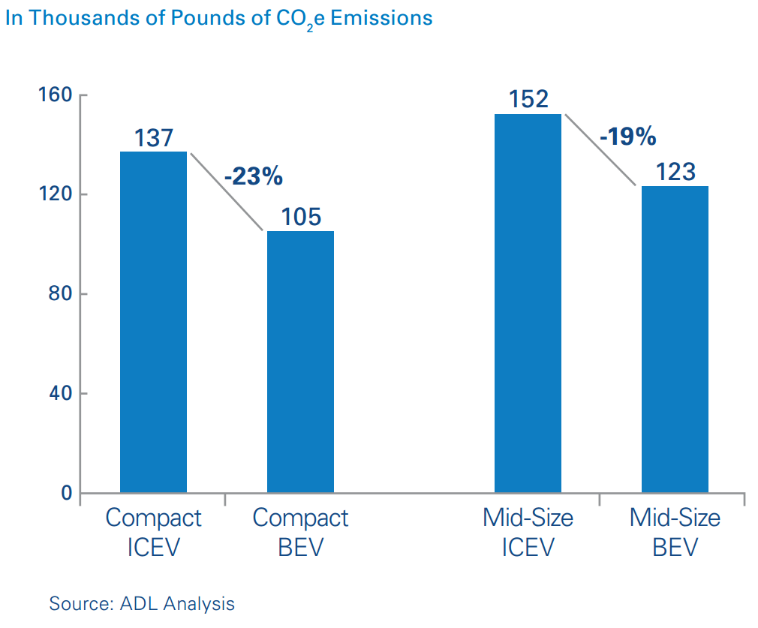
Overview of Range Extender Technology for Electric Vehicles
Range extender technology should be discussed in regard to electric vehicles. Range Extender Electric Vehicle (REEV) refers to a multi-domain engineering system that implements a range extender, or a fuel-based auxiliary power unit to extend the battery range through charging it by an electric generator. The road ability on a REEV depends on “the balance of subsystems” (Wahono, Santoso, and Nur, 2015, pp. 410).
According to Veza et al. (2020, p. 2), BEVs are characterized by low range characteristics, which contributes to customers’ anxiety and hinders market development for the technology. Range extender technology offers a solution to this issue, lowering the BEVS’ capital costs by downsizing the battery. As presented in Table 1, the battery capacity of an electric vehicle (Chevrolet Volt) equipped with a range extender covers less than 60 km driving range. In contrast, an electric vehicle without a range extender (Renault Fluence ZE) can reach up to 185 km. At the same time, the range extender in the first model can increase the total driving range to over 480 km, even though its battery capacity is 27% lower than the model without a range extender.

Furthermore, it is essential to compare the range extender EVs, EVs, and IC engine vehicles. As presented in Table 2, the driving range of EVs without range extender has a maximum of up to 210 km, while IC engine vehicles can be driven to more than 700 km. Even though the application of the range extender technology had been put off by numerous factors, many automobile manufacturers have now launched range-extended electric vehicle models.
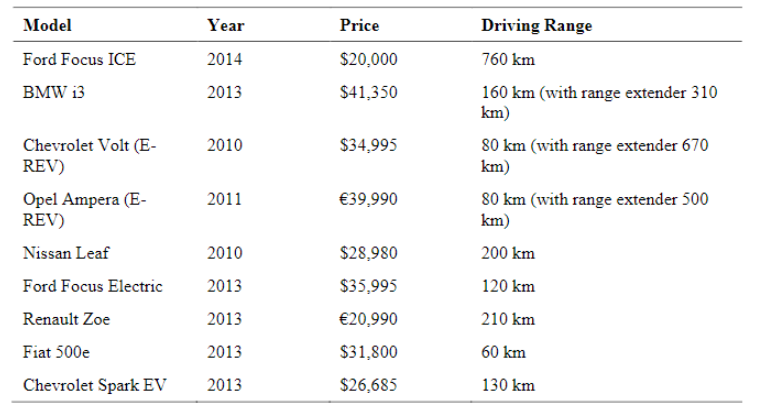
Different types of range extender technology for EVs can be discussed. They include the IC engine, microturbine, and fuel cell. For the IC engine extender, the engine and a generator are connected to the power converter. The engine will not be used if the battery is sufficient; however, when the battery is running low, the engine will be activated to generate mechanical energy. A generator then converts the mechanical energy into electrical energy that can either be stored or used by the electric motor to run the vehicle. Free-piston motors are similar to conventional IC engines except for the linear movement of the piston and connecting rod.
The microturbine works similarly to an internal combustion engine, where it converts the chemical energy into mechanical energy (Veza et al., 2020, p. 4). Since the exhaust after treatment is not required, the oil circuit can be omitted, and the unit becomes compact, light, and more affordable. Fuel cells, unlike any other range extender technology, converts the chemical energy directly into electrical energy. In this way, the necessity of mechanical energy conversion is eliminated. Moreover, compared to BEVs and fuel cell-powered EVs, it is suggested that “normal EVs with a downsized fuel cell as a range extender will be more economically attractive by 2030” (Veza et al., 2020, p. 4).
Furthermore, Low Temperature Combustion (LTC), an advanced combustion concept, can be utilized as a range extender for electric vehicles. LTC differs from conventional spark ignition (SI) combustion and compression ignition (CI) diffusion combustion concepts, offering prominent benefits, such as oxides of nitrogen (NOx) and particulate matter (PM) reduction (Singh and Agarwal, 2017, p. 11). Additionally, it reduces specific fuel consumption and can be beneficial in the implementation of automotive engines.
Vehicle to Grid (V2G) Technology and State of the Art
Vehicle-to-grid (V2G) is a concept of two-way use of electric vehicles and hybrids, which involves connecting a car to a common electrical network to recharge the vehicle. At the same time, there is an ability to send electricity back to the network to participate in electricity demand management (Mehrjerdi and Rakhshani, 2019, p. 463). Owners of cars with V2G technology have the opportunity to sell electricity to the grid during the hours when the car is not in use and to charge the car during hours when electricity is cheaper since in many countries the price of electricity depends on the time of day. It is possible to connect cars with this technology to houses and use them as uninterruptible power supplies for households.
Batteries have a finite number of charge cycles as well as an expiration date, so using vehicles as grid storage can affect battery longevity. Studies in which batteries are cycled two or more times a day have shown a significant decrease in capacity and a significant reduction in battery life (Mehrjerdi and Rakhshani, 2019, p. 464). However, battery capacity is a complex function of factors such as battery chemistry, charging and discharging rates, temperature, state of charge, and age. Most studies with slower discharge rates show only a few percent additional degradation, while one study suggested that using mesh storage vehicles could improve durability (Mehrjerdi and Rakhshani, 2019, p. 464).
Most modern battery electric vehicles use lithium-ion cells, which can achieve over 90% efficiency in both directions. Battery performance depends on factors such as charge rate, state of charge, battery health, and temperature. However, most of the loss occurs in system components other than the battery. Power electronics such as inverters usually dominate overall losses, and the overall crank efficiency for the V2G system to range from 53% to 62%. However, overall performance depends on several factors and can vary greatly.
From the perspective of vehicle-to-grid technology, EVs ‘ integration into the smart grid can be beneficial in terms of environmental and economic effectiveness. However, EV charging involves adverse impacts on the current network operation, and appropriate charging management strategies should be implemented (Yong, J. Y. et al., 2015, p. 365). In a nutshell, V2G technology refers to smart charging that results in decarbonization, energy efficiency, and electrification.
State of the art is the highest level of general development reached at a given time. In terms of vehicles, contemporary technologies enable electric and hybrid vehicle growth in the market. The integration of technologies of the automobile, electronics, and energy storage is of high importance in the modern context. The state of the art of electric vehicles should be considered in regard to technological aspects, like charging techniques and wireless power transfer. In this regard, conductive and inductive, or wireless charging system (WCS), methods can be utilized for battery charging.
According to Brenna, M. et al. (2020, p. 2539), there are stationary WCSs, which “can only be utilized when the car is parked or in stationary modes … or they can be dynamic.” In Figure 3, the onboard and the off-board chargers are depicted, showing the typical architecture of an EV. Furthermore, the principle of the static WCS for EVs is depicted in Figure 4. Both modes imply the ways of improving current technology.
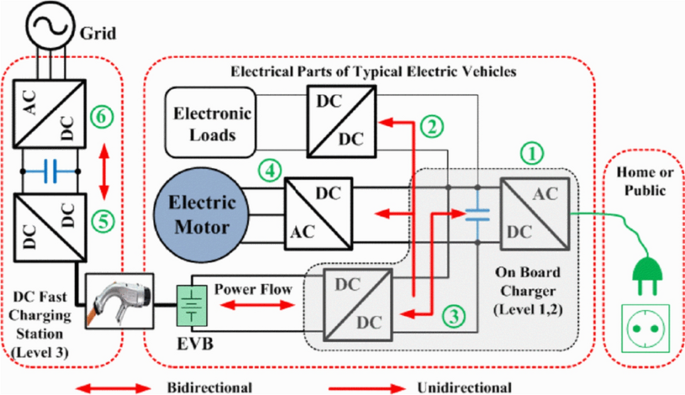
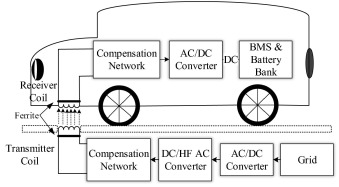
For improving the efficiency of the system, series and parallel combinations can be included on both the transmitting and receiving sides (Panchal, Stegen, and Lu, 2018, p. 924). In addition, different innovations can improve charging opportunities for EVs, such as pop-up pavement chargers, roadside street cabinets, lamp-post charging, self-heating batteries, and electrified roads.
Electric vehicle tendencies are constantly developing through the implementation of new innovations. Modernizing the battery technology use is only one of the potential ways of bringing higher value to the market while reducing cost. For improving the efficiency of the system, series and parallel combinations can be included on both the transmitting and receiving sides (Panchal, Stegen, and Lu, 2018, p. 924). In addition, different innovations can improve charging opportunities for EVs, such as pop-up pavement chargers, roadside street cabinets, lamp-post charging, self-heating batteries, and electrified roads. Constant innovations in the industry are required, along with a more developed infrastructure to accommodate the consumer demand for electric vehicles.
Part 2
A Specification for a Concept Electric Vehicle
Based on the research of the subject presented in Part 1, a specification for a concept electric car is provided in Table 3.
Table 4: Concept car specifications.
Acceleration times calculation
The calculations of the 0 to 100 kph and 0 to 160 kph acceleration times are provided in Table 5.
Table 4: Concept car specifications.
To summarize, the rapid development of innovation and new technology implementation contributes to the expansion of the electric vehicles market. A well-to-wheel efficiency, the range extender technologies, and the Vehicle to Grid technology are essential to consider in regard to the improvement of EVs. This paper provides specifications for a concept electric vehicle as well as acceleration times calculations. Overall, EV technology has developed at a rapid pace, and constant enhancements are observed.
Reference List
Song, C.H. and Aaldering, L.J. (2019) ‘Strategic intentions to the diffusion of electric mobility paradigm: The case of internal combustion engine vehicle,’ Journal of Cleaner Production, 230, pp.898-909.
Kawamoto, R. et al. (2019). ‘Estimation of CO2 emissions of internal combustion engine vehicle and battery electric vehicle using LCA,’ Sustainability, 11(9), p. 2690. Web.
Panchal, C., Stegen, S., and Lu, J. (2018) ‘Review of static and dynamic wireless electric vehicle charging system,’ Engineering Science and Technology, 21(5), pp. 922–937. Web.
Well-to-Wheel: How to better understand it (no date). Web.
Going beyond Well-to-Wheel: Life Cycle Emissions (2019). Web.
Brenna, M.,et al. (2020) ‘Electric vehicles charging technology review and optimal size estimation,’ Journal of Electrical Engineering & Technology, 15, pp. 2539–255. Web.
Battery electric vehicles vs. Internal Combustion engine vehicles (2016). Web.
Brown, S. et al. (2010). ‘Electric vehicles: The role and importance of standards in an emerging market,’ Energy Policy, 38(7), pp. 3797-3806. Web.
Hausfather, Z. (2019) Factcheck: How electric vehicles help to tackle climate change. Web.
Electric vehicle basics (no date). Web.
Veza, I. et al. (2020) ‘Potential of range extender electric vehicles (REEVS), IOP Conference Series: Materials Science and Engineering, 884(1), pp.1-6. Web.
Wahono, B., Santoso, W. B., and Nur, A. (2015) ‘Analysis of range extender electric vehicle performance using vehicle simulator,’ Energy Procedia, 68, pp. 409-418. Web.
Singh, A. P. and Agarwal, A. K. (2018) ‘Low-temperature combustion: an advanced technology for internal combustion engines.’ Advances in Internal Combustion Engine Research, pp. 9-41. Web.
Mehrjerdi, H., and Rakhshani, E. (2019) ‘Vehicle-to-grid technology for cost reduction and uncertainty management integrated with solar power,’ Journal of Cleaner Production, 229, pp. 463-469. Web.
Yong, J. Y. et al. (2015) ‘A review on the state-of-the-art technologies of electric vehicle, its impacts and prospects.,’ Renewable and Sustainable Energy Reviews, 49, pp. 365-385. Web.