Cellular solids are defined as clusters of cells, which are combined. According to Gibson and Ashby, the material in question can be seen in nature, for instance, wood or sponges are examples of cellular solids. Scientists distinguish two types of cellular solids, depending on the structure of the material. Due to the fact that this material is primarily natural and not human-made, it is difficult to trace its history correctly. However, Gibson and Ashby state that humanity has used cellular solids for more than five thousand years. Application in nature is similar to ways in which people utilize cellular solids. Gibson and Ashby state that orange peel or cork on a tree means in which nature isolates the body of a plant or fruit from the outside environment. Figure 1.1 displays foam created by humans; those are copper, mullite, and zirconia.
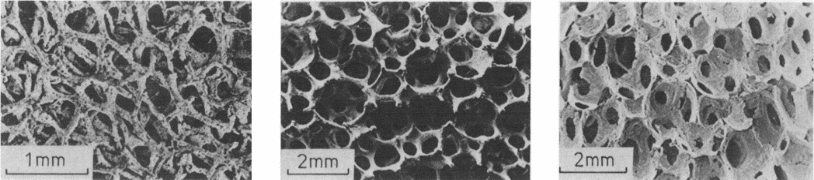
Stochastic Foams can be classified into three major groups – open-cell, closed-cell, and partially opened partially closed cells. They are considered to be a subtype of solid materials and are divided into three main categories: open-cell, encapsulated or open membranes, and partially opened or closed. Due to the specific structure of the material and its flaws, it is difficult to apply stochastic foams in manufacturing.
This type of cellular solids is also known as lattices and is characterized by well-defined unit cells. They are classified into two-dimensional honeycombs (2D) and three-dimensional polyhedrons (3D). 3D cellular solids are characterized by high energy absorption and stiffness. Thus, they have a better structure when compared to stochastic foams due to enhanced load sustainability, control of structure, and surface area density.
Cellular solids have a variety of applications in engineering due to their structure. According to Gibson and Ashby, the reason for this is in the fact that cellular solids allow optimizing weight, stiffness, and overall strength of construction. The works of Gibson and Ashby and Ashby emphasized that scientists have been interested in this material for a long time. Many modern types of research have identified new ways in which cellular solids can be used.
Baker et al. and Yamamoto et al. studied the application of cellular solids in biology by examining membrane protein and applying target protein labeling. Blake and Marangoni studied factors such as water content, oil type, and others that affect cellular solids and can be used to increase elasticity. The research can be applied in the food industry for the modification of products.
A study by Maiti et al. examined an innovative approach to human-made cellular solid materials. The authors applied 3D printing to create a polymeric foam with the required microstructure. The foam produced by 3D printing was put to an aging test together with a traditionally made one and outperformed it in compression and load retention metrics. A similar study was conducted by Hussein, who aimed at minimizing the thermal impact on cellular structures that were 3D printed. He concluded that curved beams could be used in between layers during printing. Dalaq, Abueidda, and Abu Al-Rub state that multifunctional composite materials created by 3D printing can have better damage resistance and thus be applied in architecture.
Materials made out of cellular solids can have better characteristics when compared to commonly used. Zhou, Wang, and Zhao examined cellular gradient solids, which were subjected to high-velocity impact. The critical aspect of this research was in reviewing the different density of materials and their dynamic response. Elements with a larger density gradient had higher energy absorption and smaller crushed distance. Zhou, Wang, and Zhao emphasized that in cases when the cellular layer is disrupted, the energy absorption effect is not in place, regardless of density. Rickard and Riessen state that cellular solids with low density are resistant to fire and high temperatures. Thus, they can be used as thermal resistant barriers or fire resistance panels.
New approaches to studying cellular solids were proposed over the years. Sparavigna states that a method of image segmentation through thresholding allows seeing the microcells of material in question. It enables studying their structure better and find new applications. For instance, cellular solids can be helpful in creating new materials and structures that can be applied in medicine. Gagg, Ghassemieh, and Wiria subjected porous titanium to compression testing to identify stress-strain curves.
The findings indicate that alterations applied to geometry constants can be used to adjust methods that are currently used for behavior prediction of cellular solids with high density. Dorodnitsyn and Van Damme studied cellular solids regarding their application in acoustics. The authors examined the concept of metamaterials composed of cellular solids in which cells are filled with fluids. In such a structure, the two materials interact, impacting elastic wave propagation. Such a combination of closed-cell cellular solid filled with liquid can have different practical applications.
Cellular solids can be used to create ultra-lightweight structures. According to Meza, Das, and Greer, microstructural architecture can be applied to enhance the mechanical properties of these materials. Additionally, new approaches to development demand different materials. Cheng et al. state that cellular structures can be adapted to additive manufacturing. The term describes a strategy of bottom-up development, opposite to the traditional ways. This would enable incorporating components with complex geometry and topology into a structure. Cellular solids can be used to create ultra-lightweight structures. According to Meza, Das, and Greer, microstructural architecture can be applied to enhance the mechanical properties of these materials.
References
- M. F. Ashby and R. F. Mehl, “The mechanical properties of cellular solids”, Metallurgical Transactions, vol. 14a, pp. 1755-1769, 1983.
- L. A. Baker, M. D. Elwin, A. W. van der Cruijsen, G. E. Folkers, and M. Baldus, “Efficient cellular solid-state NMR of membrane proteins by targeted protein labeling,” Journal of Biomolecular NMR, vol. 62, pp. 199-208, 2015.
- A. I. Blake, and A. G. Marangoni, “Factors affecting the rheological properties of a structured cellular solid used as a fat mimetic,” Food research International, vol. 74, pp. 284-293, 2015.
- L. Cheng, P. Zung, E. Biyikli, J. Bai, J. Robbins and A. To, “Efficient design optimization of variable-density cellular structures for additive manufacturing: Theory and experimental validation,” Rapid Prototyping Journal, vol. 23, pp. 660-677, 2017.
- A. S. Dalaq, D. W. Abueidda, and R. K. Abu Al-Rub, “Mechanical properties of 3D printed interpenetrating phase composites with novel architectured 3D solid-sheet reinforcements,” Composites Part A: Applied Science and Manufacturing, vol. 84, pp. 266-280, 2016.
- V. Dorodnitsyn and B. Van Damme, “Two-dimensional fluid-filled closed-cell cellular solid as an acoustic metamaterial with negative index,” Physical Review B, vol. 93, pp. 134302, 2016.
- G. Gagg, E. Ghassemieh, and F. E. Wiria, “Analysis of the compressive behaviour of the three-dimensional printed porous titanium for dental implants using a modified cellular solid model,” Journal of Engineering in Medicine, vol. 227, pp. 1020–1026, 2013.
- L. J. Gibson and M. F. Ashby, Cellular solids: structure and properties: Cambridge university press, 1999.
- A. Y. Hussein, “The Development of Lightweight Cellular Structures for Metal Additive Manufacturing,” 2013.
- A. Maiti, W. Small, J. P. Lewicki, T. H. Weisgraber, E. B. Duoss, S. C. Chinn, … T. S. Wilson, “3D printed cellular solid outperforms traditional stochastic foam in long-term mechanical response,” Scientific Reports, vol. 6, pp. 24871, 2016.
- L. R. Meza, S. Das, and J. R. Greer, “Strong, lightweight, and recoverable three-dimensional ceramic nanolattices,” Science, vol. 345, pp. 1322-1326, 2014.
- W. D.A. Rickard, and A. Riessen, “Performance of solid and cellular structured fly ash geopolymers exposed to a simulated fire,” Cement and Concrete Composites, vol. 48, pp. 75-82, 2014.
- A. C. Sparavigna, “Image segmentation applied to the study of micrographs of cellular solids,” International Journal of Sciences, vol. 6, pp. 68-76, 2017.
- K. Yamamoto, M. A. Caporini, S. lIm, L. Waskellcd, and A. Ramamoorthy, “Cellular solid-state NMR investigation of a membrane protein using dynamic nuclear polarization,” Biochimica et Biophysica Acta (BBA) – Biomembranes, vol. 1848, pp. 342-349, 2015.
- H. Zhou, X. Wang, and Z. Zhao, “High velocity impact mitigation with gradient cellular solids,” Compositers Part B, vol. 85, pp. 93-101, 2016.