Introduction
The past decade has witnessed some progress in the development of Pt-based catalysts for oxygen reduction reactions (ORR). In fact, some are currently being used in production on a commercial scale, specifically for polymer electrolyte fuel cells (PEFCs) in automobiles. Further developments in Pt-based catalysts were informed by the need to increase the uptake of PEFCs. It is without a doubt that oxygen reduction reaction on a Pt-based catalyst remains to be one of the essential cathode reactions in a fuel cell (Yi et al. 113). The high demand for energy, currently being witnessed, has seen fuel cells emerge as a potential alternative to traditional energy technologies. Although most Pt-based catalysts are costly, they are irreplaceable key components for fuel cells existing in acidic conditions.
As evidenced in research, Pt-based catalysts act as a major reactant element in fuel cells. This type of reaction takes place via four different electrons at the cathode. However, this process was initially hindered by multistep mechanisms until the recent development of composition-controlled Pt-based ORR electro-catalysts. Another development of Pt-based catalysts, which will be discussed later, revolves around alloying with non-precious metals, which has proven effective in optimizing the adsorption energy of Pt. Overall, the recent developments in Pt-based catalysts have been made possible by strategies such as alloying, morphology and compositional control.
Oxygen Reduction Reaction Mechanisms
The best way to understand the oxygen reduction reaction process is through the aqueous electrolyte. The process takes place at the cathode of fuel cells and occurs through the following steps. First, the oxygen molecules are diffused and adsorbed at the surface of the electrocatalysts. Secondly, the electron is transferred from the anode to adsorbed oxygen molecules. Thirdly, the weakening and splitting process of oxygen molecules to O-O follows suit. The last step is the process of removing the produced OH- ions to solutions. In other words, oxygen reduction reaction process is completed either through “a highly efficient four-electron pathway in one step or a sluggish two-electron pathway in two steps” (Zhang et al. 268). The figure below shows two adsorption types (bidentate oxygen molecule adsorption and end-on oxygen molecule adsorption) on the surface of carbon material.
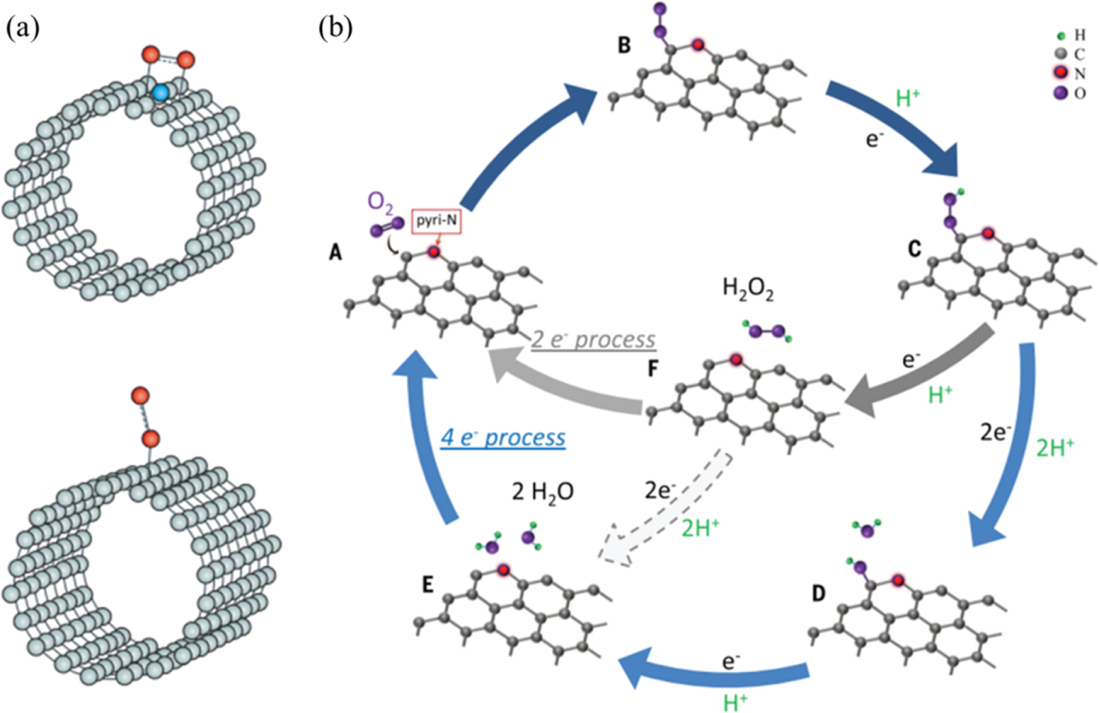
The mechanism behind Pt-Based Catalysts
Platinum is increasingly being considered as the standard catalyst for many reduction reactions. As explicated by Wang et al. in their study, platinum acts as a catalyst by accumulating oxygen atoms and allowing them to bind with carbon monoxide (CO) (see figure 2). This, in the end, leads to the creation of carbon dioxide, which is less harmful. In a different study, Xiong et al. were more interested in finding out why platinum is used over other catalysts (1976). Their findings showed that a good catalyst “should be able to split up oxygen molecules into atoms but not bind too strongly with the free atoms” (Xiong et al. 1979). Similarly, the shorter distance between atoms makes Pt-based catalysts more effective.
The main role of Pt-based catalysts is to oxidize carbon monoxide. Platinum is considered the metal of choice because it works best under oxygen-excessive conditions. For instance, platinum works well for petrol-powered vehicles, provided the exhaust gas has a balance between the reducing agents and oxidants (Kodama et al. 147). However, there is a need to use platinum in combination with rhodium because of its efficiency in reducing nitrogen oxides to nitrogen.
For Pt-based catalysts, the most recognized way of improving their activities is to double the percentage of exposed Pt sites per mass that interact with oxygen reactant. This is often achieved by doubling the number while, at the same time, preventing the blocking surface of Pt atoms. In addition to this, the binding energy found between the Pt atom’s oxygen components can be weakened by tuning the electronic structures of the Pt site.
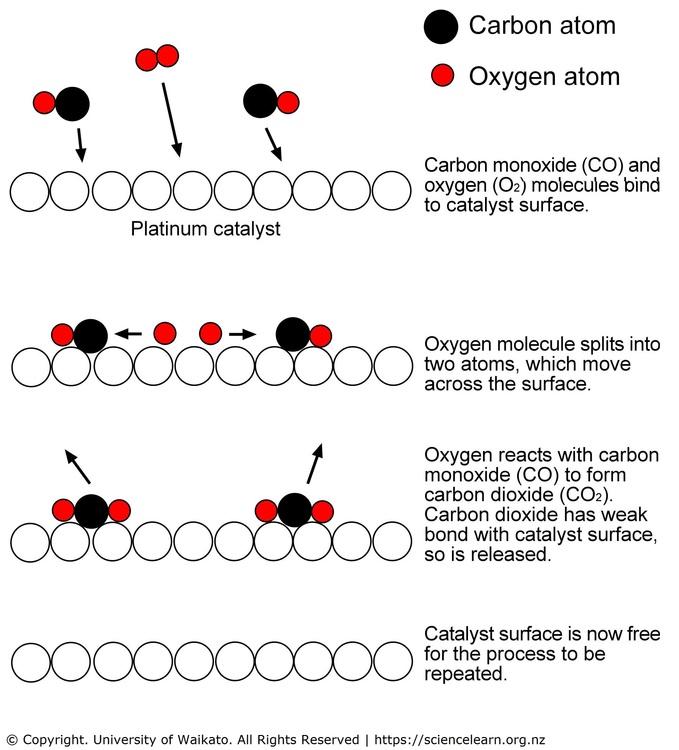
Current Development of Pt-Based Catalysts for Oxygen Reduction
Core-Shell Structured Pt-Based ORR Electrocatalysts
The past few years have seen major progress in the development of core-shell structures with the aim of improving oxygen reduction activity and stability of Pt-based catalysts. From the onset, as explicated by Zhang et al., the focus was to ensure the ligand and geometric effects were tuned spontaneously. The process started with a theoretical study in which both the stability trends and oxygen reduction activity were scanned in the materials cited in Figure 3 and 4. By using the density functional theory calculations, the researchers identified 700 core-shell with several Pt-based nanostructures based on the bonding energies (Corona et al. 244). It is evident from figure 3 that higher oxygen reduction activity corresponds to darker shades of color with low Pt metal loading. Figure 4 for stability helps paint a clear picture of why NPs colored orange appear more stable: it is due to the swapping of a core atom with a shell atom in a vacuum.
In line with the above, a major current development of Pt-based catalysts is activeIr@Pt core-shell catalyst. It consists of platinum over-layers and nanostructure effects which direct oxygen to the platinum surface and, in the process, improve the stability and activity of oxygen reduction reaction. Strickler et al. carried out an electrochemical analysis of Ir@Pt nanoparticles with multiple shell thicknesses. Their findings indicated an overall improvement in the activity and stability of the Ir@Pt catalyst (Strickler et al. 244). This led them to conclude that the activity and stability improvement of the Ir@Pt catalyst was a result of the weakening of oxygen binding to Pt surface initiated by the Ir core.
The development of Ir@Pt catalyst soon gained attraction, with scientists looking for new ways of improving on it. According to Wu et al., the catalyst could be further improved upon through a well-coordinated alignment of the “Pt shell thickness, particle size and core composition” (151). This explains why, currently, scientists are more concerned with understanding the mechanistic features of core-shell nanoparticles. In fact, scientists are already using scattering analytics for bulk compositional analysis to help characterize core-shell particles. In other words, core-shell structured Pt-based ORR electrocatalysts are concerned with how electronic and structural properties of Core-Shell can be tailored to Pt-shell thickness and core composition to improve stability and reactivity.
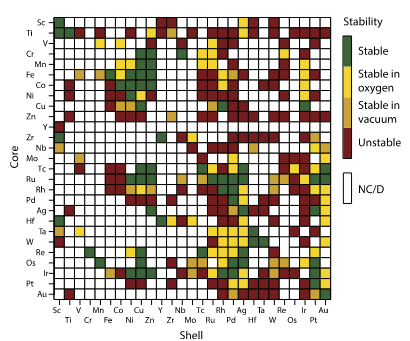
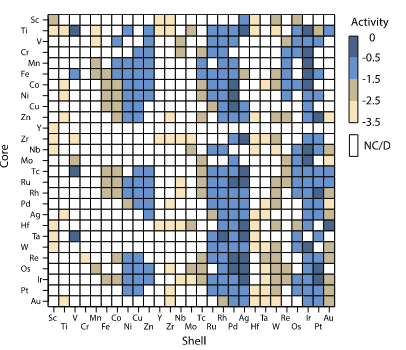
Alloying and Doping
Research has shown that alloying two or more metals may give rise to catalysts with distinct properties. Back in the early 2000s, scientists were aware that the activity and stability of oxygen reduction could be improved by alloying Pt with Ni, Mn and Co, which acted as transition metals (Wang et al. 1511). The scientists understood that the electronic structures of platinum could be improved by alloying to enhance oxygen reduction performance. In a different research, Fu et al. were interested in finding out how DFT calculation could help with understanding the function of alloying metals (102). Their findings indicated several activities of PtM alloys exhibiting similar volcano-type relationships with d-band center: it acted as a descriptor in ORR.
The above conclusions inspired the current development of Pt-based catalysts for oxygen reduction. A good example is the Yang group whose focus was to show how Pt3Ni truncated-octahedral nanocatalysts if applied well, could expose facets. The group’s findings showed an overall improvement where the particles exhibited a 4 oxygen reduction activity compared to commercial Pt/c. The findings prompted the group to embark on preparing PtM alloys, as shown in figure 4. This led them to conclude that oxygen reduction reaction can be improved through the use of Pt3Niicosahedra as a catalyst.
In Markovic’s research, the findings indicated that PtM alloys are made of a compositional segregation features which explains why they display a unique property and configuration. Markovic’s conclusions guided Shviro et al. in their research on alloy nanocatalysts (199). The researchers focused their study on the morphological and compositional development of three octahedral PtxNi1−x alloy NP. To achieve their objective, they relied on both scanning transmission electron microscopy (STEM) and electron energy-loss spectroscopy. Shviro et al. observed a “leach in the three octahedral facet centers and concave octahedral structure” (220). In the year 2020, the authors carried out an in-depth study on specific elements of degradation of Pte alloy nano-octahedra. The results indicated a further improvement in oxygen reduction, which was possible with synthesizing Pt alloy ORR electrocatalysts.
Another development in relation to alloying and doping originated from Wang et al.’s study. The Adzic group spearheaded the development of Pt/C catalyst that was stabilized by Au nanoclusters. With the use of in situ X-ray and voltammetry data, Wang et al. maintained that Au clusters improve the platinum oxidation potential and, in the process, enhance ORR stability (1511). Xia group, inspired by Wang et al.’s research, prepared “a Pd-Pt bimetallic nanostructure with Pt branches on a Pd core” through the reduction Pt salt with Pd nanoseeds (Davidson et al. 100). Their findings showed an overall improvement in ORR stability and activity at room temperature. The enhanced ORR performance was largely attributed to large surface areas.
Nanoscale Morphology-Controlled Pt-Based ORR Electrocatalysts
Since the development of Pt3Ni , scientists have made several attempts to convert single crystals to nanoparticles. More specifically, the scientist’s focus has been directed at reducing the gap between bulk crystals and nanoparticles through thin film structures. Yi et al., in their study, were more concerned with understanding the performance of ORR on Pt overlayers embedded in the Pt-Au film (113). After an extensive analysis, the authors were able to explain the effects emanating from ligand and ensemble. For credibility purposes, the authors utilized the electrochemical layer-by-layer deposition method, which showed that (sub) monolayer amounts of Pt had a high reactivity level than Pt.
The many attempts to reduce the gap finally paid off but there was a need to reduce metal usage. This saw many scientists start preparing Pt layers on multiple substrates. First, they relied on the electrochemical deposition method to prepare atomic Pt layers on transition metal nitride. The results showed a high performance: there was a significant increase in mass activity by 4 times. Another milestone was reached with the preparation of TiNiN@Pt catalysts coated with multiple Pt atomic layers. As Kim et al. observed, it revealed completely “intact core-shell structures and extremely good durability” (200). Scientists were equally interested in how ORR activity and durability improved after synthesizing octahedral [email protected] nanocrystals with an ultrathin PtNi alloy shell.
Pt3Ni nanoframes are considered to be a major development in the field of Pt-based catalysts.The preparation process incorporated the use of 3D electrocatalystic surfaces. Shviro et al. reported that the “ideal nonaframecatalyst represented by Pt3Ni (1.5) forms a more uniform Pt-skin due to its increased segregation of platinum” (190). With its open structure, Pt3Ni was found to be more reactive: the reactants could reach its internal and external surfaces. More specifically, it had a performance index of 7 which is 22 times higher compared to Pt/C catalysts. Overall, its mass activity surpassed the U.S Department of Energy’s 2020 target of 0.44A/mg Pt: its mass activity was slated at 5.7 A/mg Pt. Pt3Ni nanoframes was further modified with the addition of ionic liquid [MTBD][NTf2]. This new modification led to an overall improvement in mass activity by 32 compared to Pt/C. The addition of ionic liquid also gave Pt3Ni nanoframes higher activity without any single decay upon 1000 possible cycling.
One final development in the field of nanoscale morphology-controlled pt-based ORR electrocatalysts was reported recently. Wu et al. Showed in their work how Pt/NiO core/shell nanostructure could be used as a hydrogen gas sensor (160). First, they used wet chemistry method to synthesize Pt/NiO, which was then followed immediately by thermal annealing. The results showed a significant improvement in oxygen reduction activity of 12 Ma/cm2 and mass activity of 13 A/mg Pt. This improvement was attributed largely to a complete rhombohedral-rich surface integration of nanowires.
Overall, thermal annealing has been cited severally as the most effective synthetic strategy for developing Pt-based catalysts. As explicated by Kodama et al., “it provides a robust but effective approach to optimize structural control” (270). A good example here is seen in the preparation of Pt alloy nanoparticles by phase synthesis: this gives rise to a disordered face-centered cubic. However, when thermal annealing is introduced, Pt alloy systems tend to appear completely structurally ordered. As observed by Davidson et al., thermal annealing, when induced, brings structural ordering to Pt-based alloys (220). In addition to this, mild annealing of up to 400 °C is associated with the development of Pt-based layers through the process called surface segregation. However, when a higher temperature of more than 600 °C is used, the final result is the structural configuration of the NPs to ordering supported by phase transition. Another strategy that is currently being used is the ordering of randomly mixed alloy leads to an intermetallic compound by using a well-selected stoichiometry. The concept behind the whole process was to identify the existing relationships between quantities of reactants before and during chemical reactions. This approach has proven to be effective in influencing catalytic reactivity.
Facet-Controlled Pt-Based ORR Electrocatalysts
There is a growing interest in understanding the physical and chemical properties of Nanoscale after El-Sayed published a report describing the synthesis of cubic and tetrahedral Pt nanoparticles. One area that has been the center of focus for many scientists for the past ten years has been understanding the ORR activity of shape-controlled PGM alloy. Informed by the Pt3Ni ’s high ORR performance, the scientists prepared octahedral, icosahedral Pt-based nanostructures coated by facets and other related Pt nanoparticles. This led to the development of highly efficient and durable electrocatalysts for ORR.
In line with the above, several attempts have further been made aiming at synthesizing uniform 9 nm Pt-Ni octahedral nanoparticles by utilizing oleylamine and oleic acid as surfactants. The addition of W (CO) 6 as a representation of carbon monoxide for facet control together with benzyl as solvent has contributed a lot to understanding the reactivity state of Pt-Ni octahedral nanoparticles. This was then followed by the addition of acetic treatment to remove the surfactant. The results saw Pt-Ni octahedral nanoparticles exhibit a high ORR activity of 51 times compared to Pt/C. It was later argued that the introduction of solvent prevents a possible formation of Ni particles and, in the process, reduces the surface adsorption of surfactants. The XPS and XRD analyses further revealed a clear and well-controlled surface of the octahedral Pt-Ni nanoparticles after Ni particles were eliminated. It is this clean surface that was responsible for a high ORR activity.
Structural Ordering in Pt-based Alloy Electrocatalysts
The Pt and alloy atoms in typical Pt-based alloys tend to occupy crystallographic sites, which explains why most of them present a disordered Pt structure. The scientists were interested in finding out how best to limit the catalytic active surface sites. Another issue that needed immediate attention was the failure of the disordered alloy structure to stabilize alloy atoms and how to prevent leaching caused by high electrochemical oxidation conditions. These two issues informed the development of “structurally ordered intermetallic phases in Pt-based alloy systems”: they spearheaded an overall improvement of catalytic activity and stability (Xiong, et al. 1977). As further explicated by the authors, the Pt and alloy atoms are located at lattice points within the intermetallic face. In other words, the arrangement of alloy atoms at the lattice signals the formation of a well-controlled crystal structure. In addition to this, it is the definite composition and structure that makes ordered Pt-based alloys have high ORR performance than disordered ones.
Challenges in Development Progress
Although there have been tremendous improvements as far as Pt-based catalysts are concerned, the ORR research community still face the challenge of developing electrocatalyst with high activity and durability through scalable synthesis. In fact, researchers, for the past ten years, have been striving to address the issue. Another problem that still affects the researchers is how to reduce metal usage and enhance electrochemical stability without necessarily interfering with electrocatalytic performance. One possible solution to the problem currently being considered is the “preparation of a highly stable single atom noble metal catalyst on conductive electronic support” (Kodama et al. 140). It is also believed that a superior metal support interaction could be achieved by relying on the local catalytic environment: It has been cited severally to be a major contributor to increasing the speed of adsorption. Another possible solution to address the problem is designing core-shell catalysts with strong and compact layers of noble metals.
Another common challenge affecting the ORR catalyst development process is the lack of proper mechanisms to address the gap in ORR performance between microelectrode array (MEA) and disk electrode (RDE) measurements. Resolving the problem, would require reducing the distance/bringing closer the testing procedures and local environments. While this is the case, the researchers are yet to find a long-term solution to the mass transfer issue in the MEA devices and other related techniques.
Conclusion
The past few years have been marked by tremendous progress in the development of Pt-based oxygen reduction. As evidenced above, these developments were informed by the need to increase the uptake of PEFCs. While most of the Pt catalysts are considered costly, they are a critical component for fuel cells existing in acidic conditions. Some of the developments discussed include core-shell structured pt-based ORR electrocatalysts, alloying and doping, and facet-controlled pt-based ORR electrocatalysts. Regarding alloying and doping, research has shown alloying two or more metals may give rise to catalysts with distinct properties. This led to development of how Pt3Ni truncated-octahedral nano catalysts by Yang group. The Adzic group also joined suit with the development of Pt/C catalyst that was stabilized by Au nanoclusters.
It is the Pt/C catalyst that influenced major developments as far as Pt-based catalysts are concerned. A good example here is the development of Pt-Ni octahedral nanoparticles which exhibited a high ORR activity of 51 times compared to Pt/C. Another development came through the modification of Pt3Ni nano frames with the addition of ionic liquid [MTBD][NTf2]—this translated to a high improvement in mass by 32. However, there are several challenges that have slowed the development process of Pt-based catalysts. They include lack of proper mechanisms to address the gap in ORR performance between microelectrode array (MEA) and disk electrode (RDE) measurements.
Works Cited
Corona, Benjamin, et al. “Computational Screening of Core@ Shell Nanoparticles for the Hydrogen Evolution and Oxygen Reduction Reactions.” The Journal of chemical physics, Vol. 4, no. 15, 2016, pp. 244-708.
Davidson, Edwin, et al. “Ultrafast and Sensitive Colorimetric Detection of Ascorbic Acid with Pd-Pt Core-Shell Nanostructure as Peroxidase Mimic.” Sensors International, Vol. 1, no. 12, 2020, pp. 100-231.
Fu, Chengrui, et al. “DFT Calculations: A Powerful Tool for Better Understanding of Electrocatalytic Oxygen Reduction Reactions on Pt-Based Metallic Catalysts.” Computational Materials Science, Vol. 2, no. 17, 2019,pp. 109-202.
Kim, Jun, et al. “Highly Stable Pt‐Based Ternary Systems for Oxygen Reduction Reaction in Acidic Electrolytes.” Advanced Energy Materials, Vol. 10, no. 41, 2020, pp. 200-249.
Kodama, Kensaku, et al. “Challenges in Applying Highly Active Pt-Based Nanostructured Catalysts for Oxygen Reduction Reactions to Fuel Cell Vehicles.” Nature Nanotechnology, Vol. 1, no. 16, 2021, pp. 140-147.
Shviro, Meital, et al. “Morphological, Structural, and Compositional Evolution of Pt–Ni Octahedral Electrocatalysts with Pt‐Rich Edges and Ni‐Rich Core: Toward the Rational Design of Electrocatalysts for the Oxygen Reduction Reaction.” Particle & Particle Systems Characterization, Vol. 3, no.36, 2019, pp. 180-442.
Strickler, Alaina L., Ariel Jackson, and Thomas F. Jaramillo. “Active and Stable Ir@ Pt Core–Shell Catalysts for Electrochemical Oxygen Reduction.” ACS Energy Letters, vol. 1, no. 2, 2017, pp. 244-249.
Wang, Xiaoqian, et al. “Review of Metal Catalysts for Oxygen Reduction Reaction: From Nanoscale Engineering To Atomic Design.” Chem, Vol. 5, no. 6, 2019, pp. 1486-1511.
Wu, Chun-Han, et al. “Pt@ NiOCore–Shell Nanostructure for a Hydrogen Gas Sensor.” Journal of Alloys and Compounds, Vol. 8, no. 14, 2020, pp. 151-815.
Xiong, Yin, et al. “Revealing the Atomic Ordering Of Binary Intermetallics Using In Situ Heating Techniques at Multilength Scales.” Proceedings of the National Academy of Sciences, Vol.1, no. 16, 2019, pp. 1974-1983.
Yi, Shijie, et al. “Recent Progress of Pt-Based Catalysts for Oxygen Reduction Reaction in Preparation Strategies and Catalytic Mechanism.” Journal of Electroanalytical Chemistry, Vol. 8, no. 48, 2019, p. 113-279.
Zhang, Changlin, et al. “A Review of Pt-Based Electrocatalysts for Oxygen Reduction Reaction.” Frontiers in Energy, Vol. 3, no. 11, 2017, pp. 268-285.