Structural organization of ovarian surface epithelium (OSE) in non-mammals
Most of the classical ovarian histological work was devoted to the species of teleosts, amphibians and reptiles. The germinal epithelium was a preferred term over OSE because this outer ovarian epithelium was assumed to be the source of germ cells in embryos and adults, and besides this, pre-follicular and granulosa cells that encircled in different shapes and sizes the oogonia, oocytes and ova, also derived from this epithelium. Many external factors were detrimental to the differentiation of germinal epithelium-derived- germ cells to either spermatogonia or oogonia. Accordingly, the organization of germinal epithelium into follicular structures also differed in the male or female gonads. Hence, to understand the germinal role of surface epithelium in mammals, including humans, it is necessary to gain an insight into this tissue in non-mammalian species. Duke (1978) compiled the classical histological data on variations in size, shape and pattern of germinal epithelial (now referred to as OSE) cells in different taxonomic divisions of non-mammalian vertebrates.
In teleost fishes (Fig. 1), the ovary develops folds and grooves called ovigerous folds, resulting in the formation of a central endovarial cavity lined with OSE. Another secondary change results from the fusion of ovarian folds with the dorsolateral body wall, in which ovigerous folds are enveloped with surface epithelium by an ovarian wall of the parovarian cavity. In brook lamprey (Petromyzone marinus) the OSE cells of developing ovary varied from squamous to cuboidal in shape. The cuboidal shape was often associated with the proliferation of satellite or follicle cells of adjacent germ cells. Development of ovary in two elasmobranches genera, Scyllium and Raja, led to an observation that proliferation of thickened OSE in underlying mesenchymal “core of stroma” formed a rudimentary gonad. In basking shark (Cetorhinus maximus) the OSE layer is invaginated so that the ovary is a hollow organ called pockets, which are broken up in innumerable fine tubules that join to form progressively larger tubes, opening to the surface in the pockets. Thus, for all practical purposes, OSE lines the interior of the ovary and ripe ova are released into the internal tubules which open into coelom by making way through the pockets. Many teleosts have hollow ovaries with ovigerous folds enclosed within the ovarian cavity by the ovarian wall. During gestation in viviparous teleosts like Neotoca bilineata, OSE undergoes marked changes like the cells of original flattened epithelial cells of OSE transform to columnar and swollen at late gestation stage. This epithelium is the surface epithelium before the formation of the ovarian cavity, which also serves as the uterus. Since the epithelial-lined pockets of ovigerous folds also contain oogonia, it was postulated that germ cells are derived from surface epithelium. Despite the controversy on the origin of germ cells, the fact remains that there is an intimate positional relationship between epithelial pockets of ovigerous folds and germ cells in viviparous teleosts. More recently OSE organization in oocyte development was investigated in swamp eel, Synbranchus marmoratus (Ravaglia & Maggese, 2002). There is a “U-shaped” ovigerous fold within the ovarian wall, and between the fold and the ovarian wall there is a cavity, the ovarian lumen. Oogonia are distributed homogeneously along the inner surface of the OSE, which covers the ovarian cavity, and are located between epithelial and/or pre-follicular cells. In fact, these epithelial cells become pre-follicle cells when are associated with oogonia. Surface epithelial and pre-follicular cells were separated from underlying lamellar stroma with a basement membrane. In bony fishes, the OSE is spread along the ovarian lamellae and is composed of somatic cells, and the germ cells can regenerate into ova (Parenti & Grier, 2004). Sex reversal is a unique feature in protogenous species and the same OSE destined to produce oogonia starts to produce male germ cells developing into sperms.
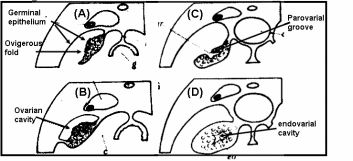
The ovary of amphibians is covered by a flattened layer of epithelial cells, in which pockets of oogonia at scattered intervals are located. At all stages, oocytes are covered with three cellular layers; the outermost simple squamous surface epithelium, beneath this a fibroblasts layer and the innermost layer of follicle cells encircling oocytes (Sretarugsa et al. 2001). Ripe ova are released in coelomic cavity by rupture of OSE and some other intervening tissue. The OSE of a viviparous lizard, Xantusia vigilis, consists of avsingle layer of flattened epithelium with two regions where oogonia and surface epithelium form small masses protruding inwards. In box turtle, Terrapene carolina, oogonia and epithelium clusters as the “germinal ridges” at the periphery of the ovary, and in musk turtle, Sternotherus odoratus, OSE proliferates in the cells that give rise to the epithelial component of sex cords and stromal elements. The ovaries of reptiles are lobulated with quite compact germinal centers. The germinal center in reptiles refers to the collection of oogonia and/or oocytes associated with the OSE. One unique feature in many oviparous reptilian species is temperature and hormonal dependence of gonadal sex differentiation of the eggs (Pieau & Dorizzi, 2004). In turtles at low-temperature OSE proliferates and develops into epithelial chords (sex-chords) in the inner part of male gonads, whereas at higher temperatures OSE lines the interior of lobules and encapsulates the female germ cell for oocyte development. The ovary of marsupials and eutherian mammals is suspended from the dorsal body wall by a mesentery, the mesovarium. The ovary is covered with a peritoneal layer except for the site at which it is attached to the mesovarium. OSE comparison is given in Fig. 2.
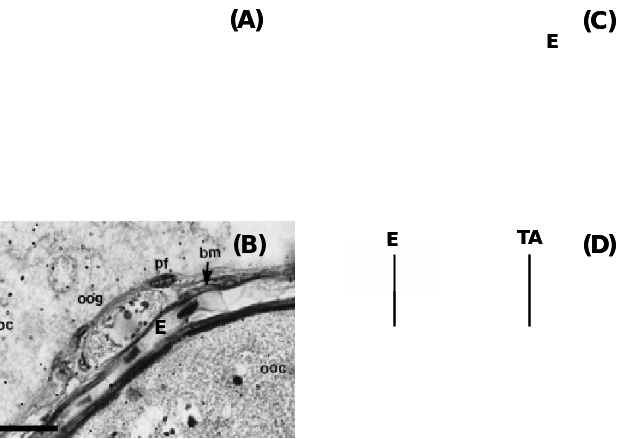
(A) Teleost fish Fundulus grandis showing the positions of oogonia (OG) and pre-follicle cells (PFC) within the epithelium (E) and beneath are the prethecal cells (PT) covering extravascular space (EVS) and primary growth oocytes (PGOC), (B) Another teleost, Synbranchus marmoratus showing oogonia (oog) covered with prefollicular cells (pf) located underneath the epithelium (E) separated by a basement membrane (bm), (C) Amphibian, Rana tigerina, showing epithelium (E) and underneath theca layer (TL) and follicle cells (FC) covering Vitelline envelope (VE) of oocytes, and (D) Ovine epithelium (E) above basal lamina (arrow) and underneath tunica albuginea (TA). Taken from Murdoch & McDonnel (2002), Parenti & Greier (2004), Ravaglia & Maggese (2002) and Sretarugsa et al. (2001).
Structural organization of OSE in mammals
Mammalian OSE is comprised of predominantly club-shaped squamous to cuboidal cells covering the entire internal ovarian surface, and usually do not undergo complex structural organization (Auersperg et al. 2001). According to Duke (1978), insectivores like Blarina brevicauda and Sorex araneous have thin, inconspicuous OSE, whereas Scalopus aquaticus has pseudostratified columnar epithelium of ca. 20 µm thickness. Oogonia, oocytes and transitional stages of ova associate with OSE layer in diverse species, but in the higher mammals the OSE is not in contact with different differentiating stages of follicles except during ovulation. In many species, the proliferation of epithelium into the ovarian cortex has been reported, and mostly they generate epithelial crypts and inclusion cysts (Fig. 3). It was found that number of proliferations was correlated with the estrous cycles. In anestrous water strew, Neomys fodiens, the thickening of the OSE layer was restricted to certain areas of ovarian surface and it was not related to this seasonal activity. Moreover, the occurrence of testis-like cords in ovaries of Sorex vagrans, the vagrant strew was attributed to the proliferation of surface epithelium. The most noticeable proliferative activity was recorded for cords and/or tubules comprised of OSE into the cortex and associated oogonium and oocyte formation in several species of prosimian primates. In a ripe follicle (antrum), the cuboidal cells which make up the OSE are loosely attached to the non-discrete basal lamina at the surface of the ovarian tunic. Cuboidal cells also make up the inner lining of the surface crypts and inclusion cysts in the ovarian cortex. These cells contain large, indented nuclei and their cytoplasm has numerous mitochondria, occasionally lipid-like (mucin) inclusions and sometimes contains lysozomes. Prominent microvilli extend from the peritoneal surface of the cells. As an exception, in rodents, the inclusion cysts and crypts are not found despite repeated and multiple ovulations and OSE monolayer persist throughout the ovulation cycle (Bukovsky & Caudle, 2008).
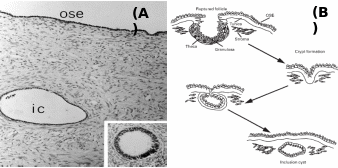
Inset: Inclusion cyst undergone metaplastic changes. (B): Stages of post-ovulatory inclusion cyst formation – ovarian surface repair by OSE forming crypts, which penetrate together with tunica albuginea into cortex to form inclusion cysts. Taken from Auersperg et al. (2001) and Ghahremani et al. (1999).
Embryonic origin of OSE cells in mammals
Embryonic development of human OSE was elaborately described by Auersperg et al. (2001). The mesodermally derived epithelial lining of the intraembryonic coelom is the future OSE. In the embryo, it covers the presumptive gonadal ridge and proliferates and differentiates to form gonadal blastema. Within 10-20 weeks of gestation the flat-to-cuboidal simple epithelium with inconspicuous and fragmentary basement membrane changes to multistratified, papillary epithelium with the well-defined basement membrane. The cells exhibit short microvilli and wide intercellular cytoplasmic projections. Moreover, the nuclei show irregular invaginations marked with pleomorphism and prominent nucleoli, indicative of high proliferative activity. Coelomic epithelium in the pelvic cavity is a source of gonadal OSE and extraovarian mesothelium but despite having identical environmental and hormonal conditions prevailing in the pelvic region, there are characteristic differences in OSE and other epithelia. For e.g. expression of CA125, a surface glycoprotein of unknown function, in an adult is localized in oviductal, endometrial and endocervical epithelium and some extraovarian epithelia, but not in the OSE. Therefore, either OSE though originated from coelomic epithelium never acquires this differentiation marker or loses it in early development. Surprisingly, CA125 is expressed in tumorigenic OSE suggesting that the original coelomic characteristic was retained by OSE but only under pathological conditions. It is believed that in humans fetal OSE is the source of at least a part of the ovarian granulosa cells. It was shown that until the 8th month of fetal life epithelial cells furnish the granulosa cells. The epithelium invaginates as ovarian chords and penetrates deep in the ovarian cortex where it gives rise to the granulosa cells in the primordial follicles, which get detached from the surface epithelium and subsequently degrade off (Fig. 4). Besides its role as a progenitor of granulosa cells, the coelomic epithelium also protrudes inwards in the vicinity of the presumptive gonads to give rise to Müllerian (paramesonephric) ducts, which constitute the epithelia of oviduct, endometrium, and endocervix. In species with ovulation fossa, the cortex constituted by modified epithelium migrates into the medullary portion of the ovary during the post-natal period. As the underlying basement membrane and other barriers are still underdeveloped the epithelial cells freely migrate into the underlying tissue and intermix with its cells.
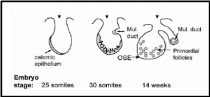
The OSE hardly exhibits proliferative activity but sometimes this can be seen in early postnatal ovaries, especially in those cases in which the unenclosed oocytes are still close to the OSE layer. In fetal canines, the OSE cells form chords and tubules which protrude deep into the stroma. Many of them even lose contact with the surface. Oocytes come to lie in these chords, and even small follicles develop in this tissue itself. At times, degenerating oocytes and follicles are found in surface tubes and chords, suggesting a mechanical role of OSE in the removal of degenerating ovarian structures. In a fetal guinea pig, the OSE cells proliferate to form multiple layers and send chords into the ovary for a brief period at the time when folliculogenesis is in progress. It was suggested that some of the oocytes in the outer part of the cortex receive cells from epithelium as follicles are developed. In the fetal mouse ovary too, a connection between OSE proliferation and folliculogenesis in the central part of the cortex was established, but the formation of follicle was not dependent on the presence of OSE.
Dynamic changes in mammalian OSE structure and function
Auersperg et al. (2001) further describe the OSE organization in adult ovaries. OSE cells are held together by zona occludens along their lateral surfaces. Several typical proteins are produced by OSE cells which are markedly different from the other extraovarian epithelia. For e.g. they express mucin antigen MUC1, 17β-hydroxysteroid dehydrogenase and cilia proteins besides the intercellular adhesion proteins viz. desmosomes, several integrins and cadherins. In humans, mouse and porcine, E-cadherin is a typical calcium-dependent adhesion protein produced in resting surface epithelia of ovary, oviduct, endometrium and endocervix. Another adhesion protein, N-cadherin, is found in the granulosa cell lining of the growing follicles. It has been suggested that when surface epithelial cells undergo columnar shape due to metaplastic or neoplastic differentiation, particularly in inclusion cysts and crypts, the N-cadherin starts to co-express along with E-cadherin. Another form of adhesion protein, P-cadherin is also typical of Müllerian origin epithelia, but is absent in resting OSE, and occasionally expresses in adenocarcinoma cell lines derived from cancerous OSE cells. It appears that N-/P-cadherins are induced during OSE neoplastia. The OSE is separated from the ovarian stroma by a basement membrane and, underneath, by a dense collagenous connective tissue layer, the tunica albuginea. A unique feature of OSE distinct from the other epithelial layer is its fragile attachment with the basement membrane that can be easily removed using mechanical means.
The most plausible function of OSE is to transport nutrients, hormones and minerals from the outer peritoneal cavity and to take part in tissue remodeling required for repair at the sites ruptured due to ovulation. OSE also helps in follicle rupture and release of the oocyte by secreting lysosomal proteolytic enzymes. It is possible that OSE secreted substance alters the underneath tunica albuginea and prior to ovulation makes it thin. Synthesis of both epithelium (re-epithelization) and connective tissue-like components of the extra-cellular matrix may contribute to the rejuvenation of the ovarian cortex. Since connective tissue fibroblasts are responsible for wound healing in diverse tissues and in cultures OSE cells maintain both epithelial and fibroblast mesenchymal characteristics it is reasonable to believe that OSE also regenerates the damaged stroma constituted mostly by mesenchymal cells. Moreover, it has been shown that these cells also share some characteristics of regenerative smooth muscle cells, like they express actin. Convolutions of OSE and formation of clefts and inclusion cysts may lead to shrinkage of ovaries at old age. Further any mechanical damage due to surgery may hinder passage from the peritoneum, and the cells then acquire the capacity to replenish the damaged portion.
The epithelial-mesenchymal transition has a capacity towards wound healing in diverse tissue types (Lee et al. 2006), and there exists a signaling mechanism for such transition. Often epithelium is a sheet of a uniform array of monolayer cells. Epithelial cells are adhered together tightly and exhibit polarity, i.e. different visible characteristics of apical and basal surfaces. Mesenchymal cells on the other hand neither exhibit regimented structure nor have tight intercellular adhesion. These cells have slightly elongated and disorganized structures and are irregular in shape. Mesenchymal cells can also leave their positions and migrate deeper into the ovarian cortex. Normally epithelium to mesenchymal transition requires alterations in morphology, cell architecture, adhesion and mobility (Fig. 5). Taking mammary cells as a model, a signal transduction pathway was proposed for such transition (Fig. 5), operational also in the OSE. It was shown that in the cytoplasm, matrix metalloproteinase-3 (MMP-3) first cleaves E-cadherin, and consequently produces reactive oxygen species and activates in the nucleus a transcriptional factor, Snail 1. Snail 1 represses the E-cadherin gene expression, thereby further lowering the cellular level of this adhesive molecule. Notwithstanding, an expression of another adhesive molecule, N-cadherin, is activated most likely through Snail 1 mediated transcriptional control. N-cadherin is four-times weaker adhesive than E-cadherin, and these cells can not sustain the intercellular adhesion. Several other epithelial-mesenchymal transition (EMT) proteins, like vimentin, are also up-regulated by the MMP-induced Snail 1. Moreover, there are other pathways operative in conjunction with the MMP pathway which collectively enables this kind of cellular transition. One of the pathways is through integrin adhesion protein, present over the cell surface, which activates through integrin-linked kinase (ILK) the Snail 1 mediated up-regulation of EMT proteins. It was further revealed that the chain of events leading to EMT is governed by the epidermal growth factor (Ahmed et al. 2006). At the moment it is not clear whether similar pathways are operative for the OSE EMT though it is likely, given the fact that MMP’s, E-cadherin and integrins are highly expressed in these epithelial cells when they are in squamous and flattened shape, and N-cadherin is expressed in the columnar cells in the clefts and inclusion cysts.
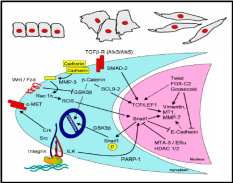
Inter-relationship between OSE layer and underneath stroma
It has been shown that EMT of OSE cells may be a mechanism to repair the perturbed stromal components and also tunica albuginea following every round of ovulation. Besides, OSE also helps in the degradation of tunica albuginea and underneath theca thereby weakening the follicular wall. In frogs and sheep scrapping off the OSE layer hinders ovulation. Hence, this layer facilitates ovulation and post-ovulatory repair. Murdoch & McDonnel (2002) put forth a model based on observations in estrous ewes, humans, mice and pigs that explains how OSE interacts with the connective tissue and follicular wall. As shown in Fig. 6, the most notable actions are collagen breakdown and cellular death of the preovulatory follicle through apoptosis and inflammatory necrosis. Gonadotropins circulated in the blood of capillaries and venules towards theca interna stimulate secretion of urokinase-type plasmnogen activators (u-PA) from within the OSE cells directed towards tunica albuginea. Since OSE cell surface LH receptors are up-regulated post-estrous due to oestradiol produced from the pre-ovulatory follicle, it is obvious that the gonadotropin effect on u-PA synthesis and secretion would be under follicle’s control. Besides, coinciding with this time there is also LH surge in higher mammals. Localized accumulation of u-PA triggers conversion of zymogenic plasminogen to a serine protease, plasmin. Unlike tissue-type plasminogen activator (t-PA), u-PA has no role in blood fibrinolysis. Plasmin synthesis occurs in the follicle’s apical hemisphere and conjoined tunica albuginea. Plasmin, in turn, activates local collagenases and releases tumor necrosis factor-α (TNF-α). The cytokine TNF-α is localized to the thecal endothelial cells of the preovulatory follicle. TNF-α binds to almost any cell, and its action is mediated through activation of TNF-α receptor-R1 (TNFR1) which is constitutively present on the cell surface. Upon binding to the target cells, it can evoke a cascade of proteolytic responses leading to apoptotic (internucleosomal) DNA fragmentation and cellular disintegration. TNF-α can also activate the transcription of zinc-containing MMP-1 and MMP-2 collagenases. Moreover, TNF-α also initiates microvascular coagulation and associated inflammation and necrotic cellular death. MMP’s are synthesized by fibroblast cells in the stroma and the steroidogenic granolosa cells of follicles. All these factors collectively degenerate coelomic epithelial layer and follicular granulosal wall at the junction to facilitate ovulation. Basement membranes on which OSE cells and the follicular granulosa cells rest are composed of type IV collagen. The follicular wall is held up by collagen I. Mammalian collagenases belong to a group of metalloproteinases (MMP) that degrade collagen. Accumulation of plasmin directly correlates with collagenase activity and with collagen degradation at follicular wall and basement membranes. After the gondotropin-induced u-PA release from OSE, there is increased activity of apoptosis (plasma membrane phosphatidyl-serine translocation, internucleosomal DNA fragmentation) in the region of surface epithelium in proximity to follicle, adjacent tunica albuginea and apical follicle wall. Apoptosis is followed by cellular necrosis, extravasation of blood cells and vascular tissue degeneration. In fact, at the time of ovulation in neither OSE nor the granulosal epithelium was found to be intact.
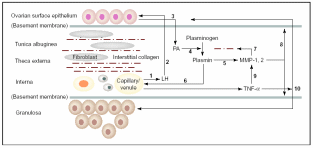
Thecal vascular LH (1) delivered to OSE (2) stimulates secretion of u-PA (3) that converts interstitial plasminogen to plasmin (4), activates collagenase MMP’s (5) and stimulates TNF-α release (6). Collagenases disrupt theca and TA (7) and promote digestion of basement membrane (8) and granulosa cells via MMP, which eventually ruptures follicle apex (10). Taken from Murdoch & McDonnel (2002).
Curry & Osteen (2003) extended this work on gonadotropin-induced MMP’s and revealed that numerous endogenous MMP inhibitors, called tissue inhibitors of metalloproteinase (TIMP), adds to the localized control of collagen degradation towards the ovarian surface. In the rodent ovary, besides MMP-1 and -2, MMP-9 and 13 and a membrane-type MMP (MT-MMP) also exist. In mouse, MMP-2 and in rat MMP-9 are constitutively synthesized while the others are stated to be under LH control. In bovine model too, MMP-2 was found to be constitutively expressed and in primates, MMP-1 was considered not influenced by gonadotropins. Furthermore, a few of the above and some unrelated MMP’s are also present in the follicular fluid and are released pre-ovulatory to act upon apical granulosa cells and collagenous membranes. In conjunction with the preovularoty increase in MMP’s, in rodents, ewe, bovine, primate and human, LH surge also induces TIMP’s in order to counter the excess collagenases action. It has been shown that a parallel up-regulation of MMP’s and their inhibitors, TIMP’s by gonadotropins in the same vicinity is a mechanism to maintain proteolytic homeostasis. Thus, the periovulatory increase in TIMP’s may act to precisely coordinate the actions of MMP’s, regulating the location and extent of ECM remodeling of the follicular apex during the ovulatory process. Alternatively, the stimulation of TIMP expression and activity may play an autocrine/paracrine role in ovulatory processes involving cellular proliferation, differentiation, or steroidogenesis. Further, it was shown that a majority of TIMP’s are produced in the basal cells of the follicle and protect the distal granulosa layer from MMP-collagenase activity, whereas the unprotected apical region gets degraded.
A fraction of OSE is constituted by stem cells
Periodic ovulation drastically damages the surface epithelium which gets rejuvenated by way or repair of the epithelial wound. The cyclic and repeated disruption and repair of the OSE with complex remodeling has led to a belief that there exists a population of somatic stem/progenitor cells within the OSE layer responsible for sustained wound healing. Somatic stem cells are just the normal tissue cells with an ability to renew themselves by asymmetric division, and thereby they produce a set of daughter cells committed to rectifying the damage by regeneration and repair. Such committed repair stem cells have been identified in a variety of tissues like skin, hair follicle, mammary gland and intestine. Parrot, Kim & Skinner (2000) investigated the expression of markers specific to somatic stem cells in the OSE layer of humans and sheep. Growth factor kit ligand (KL) and its tyrosine kinase receptor, c-kit are typical stem cell factors. KL expression was detected in granulosa cells and theca cells in the mouse. Because granulosa cells and OSE have a common cellular lineage, it was expected that OSE cells would also express these proteins. Many germ cells like melanocytes, mast cells, hematopoietic cells of the myeloid, erythroid and lymphoid cell lineages initiate their developmental program and differentiate in response to KL. Both human and bovine OSE cells exhibited positive staining with antibodies specific to KL and c-kit. KL-mRNA was also detected in OSE and to a lesser extent in bordering stromal cells, which indicates that both the epithelial and mesenchymal cells are equally capable of expressing the stem cell-specific genes though the proteins are largely present in the epithelial cells. These proteins may diffuse to the adjacent stromal mesechyma. Even the cultured OSE cells express KL-mRNA. An autocrine action of KL was proposed for ovarian epithelial proliferation surrounding the post-ovulatory wounded site responsible for re-epithelization. As KL marker was also recognizable in normal OSE cells away from ovulation site as also in the cultured cells, it was assumed that KL and c-kit may be involved in regular growth of OSE rather than a special role during re-epithelization.
In a recent study (Szotek et al. 2008) in a mouse model, several proliferative markers were used to distinguish the quiescent population of OSE cells from highly proliferative cells surrounding the post-follicular wounded region in the OSE layer. It was found that the epithelial cells, besides expressing usual epithelial marker proteins viz. cytokeratin, E-cadherin etc., also expressed vimentin, which is an epithelial-mesenchymal marker. A significant result was that two nuclear proliferation markers, bromo-dUracil (BrdU) and histone 2B green fluorescent protein (2HB-GFP) were retained by some quiescent cell populations up to four months, while the other population within the same tissue rapidly lost the markers in a short period. With high-resolution confocal microscopy, these authors demonstrated a possibility of asymmetric division of coelomic epithelial cells, which is highly characteristic of stem cells (see later). The conclusion was that those cells which lost the markers were highly proliferative somatic stem cells showing asymmetric division, and were distinct from the OSE tissue-specific cells that were not so much dividing and as a result maintained the nuclear markers for longer periods. In other words, within the otherwise morphologically identical cell types, there exist two populations of cells, of which one is constituted by the wound healing highly proliferative somatic stem cells.
The mechanism of division to somatic cell and the fate of the daughter cells in the populations of several epithelial cells have been reviewed by Morrison & Kimble (2006), and these models can be directly applied to the fate of the daughter cells derived from the division of the stem cells of mammalian OSE. The authors have proposed that stem cells are defined due to their ability to derive more stem cells by utilizing their property known as “self renewal”, and their ability to produce cells that differentiate. To accomplish this task, the stem cells use a strategy of asymmetric cell division, whereby they produce one daughter cell with a stem cell fate (“self renewal”) and the other that can differentiate. The one-to-one renewal of stem cells does not explain how the stem cells can increase their number, though this explains the totipotency of stem cells, an attribute quite relevant for differentiation to other cells. To complement the authors also claimed that stem cells also undergo symmetric cell division to self-renew their population and to generate differentiated progeny. In the normal course, a stem cell divides into exactly identical two daughter cells and this attribute renews the population. But this attribute does not explain how the differentiated cells are produced. Hence, it was further proposed that a pool of stem cells do not self renew, rather produce two differentiated daughter cells capable of generating cells different from the progenitor stem cells. In principle, stem cells can rely on either completely symmetrical cell division thereby fulfilling the requirement of self-renewed cells and differentiated cells, or can combine both symmetric and asymmetric divisions depending upon how the requirement swings. These models are sufficient to assess how epithelial stem cell divisions can rejuvenate themselves and/or generate newly differentiated cells viz. germ cells and granulosa cells. There are both intrinsic and extrinsic factors governing asymmetric cell division and are also the external factors regulating the fate of differentiating daughter cells. Asymmetric portioning of cell components like spindle fibers during mitosis that decides the fate of divided cells is an intrinsic factor, whereas asymmetric placement of daughter cells relative to external cue represents an extrinsic factor. Symmetric stem cell division is observed mostly during wound healing and regeneration. As depicted in Fig 7, a symmetric cell division in the plane of epithelial monolayer would yield daughter stem cells orienting within the same layer, but once the division occurs asymmetrically perpendicular to the epithelial monolayer, differentiated cells would make another layer atop the basal epithelial layer. In healthy adult’s injury cells can be lost from both the layers and then symmetric division would regenerate lost stem cells while asymmetric division would cover the gap of differentiated cells. In any case, homeostasis is established between the two patterns of divisions, and therefore a steady population of renewed stem cells and differentiated cells are always present in most epithelial layers. If for any reason the homeostasis is collapsed such that either symmetric or asymmetric division is favored, the event becomes deleterious and can initiate cancerous growth.
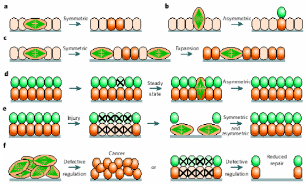
(a) Planer symmetric division in basal epithelial stem cells give identical daughter cells, (b) asymmetric perpendicular division yields differentiated cells, (c) routine symmetric division to pool-up the stem cells, (d) but it also maintains another differentiated cells’ pool. (e) Loss of any pool of cells from injury is re-modulated by symmetric/asymmetric division, and (f) defect in a switch between divisional patterns give rise to cancerous stem cells. Taken from Morrison & Kimble (2006).
Can OSE stem/progenitor cells be a source of primordial follicle germ cells?
In one of the most exciting yet controversial investigations, functional mouse sperms and oocytes could be derived in vitro from somatic cell lines (Bukovsky et al. 2004). Extending the work in humans, it was proposed that mesenchymal cells (fibroblasts) in tunica albuginea are the bipotent progenitors for both granulosa cells and germ cells. According to a model shown in Fig. 8, the fibrous connective tissue, tunica albuginea, can differentiate into OSE cells by mesenchymal-epithelial transition, and segments of OSE invaginates within the ovarian cortex to form epithelial chords which fragment further to epithelial nests and descends down inside the lower ovarian cortex. These epithelial nests lie in proximity to primary follicles consisting of one/two layers of granulosa cells encircling oocytes covered by a homogenous glycoprotein layer called zona pellucida (ZP) (ZP+ oocyte). Epithelial cells of fragmented chords are suggested to be the source of epithelial cells of primary follicles. These epithelial cells are the presumptive granolosa cells of primary follicles. Moreover, these epithelial cells also retain the embryonic structure of surface epithelium and they appear to be an alternate source of germ cells, which eventually differentiate into oocytes and associate with the granulosa cells to form new primary follicles. The stem cells of the coelomic epithelium overlaying the tunica albuginea undergo asymmetric cell division, giving rise to amoeboid ZP+ germ cells. These germ cells then symmetrically divide and descend into the ovarian cortex either through epithelial invaginations viz. crypts and inclusion cysts or they pass through the cortical intravascular system. Here most likely meiotic division takes place to produce oocytes that are picked up by the epithelial nests. The eventual development proceeds through granulosal conversion of epithelial cells, and by so-called “ova-in-ova” Balbiani body formation which ultimately develops into the primary follicle. As the germ cells enter the blood vessels in order to be transported to the epithelial nests, they can as well migrate to the peripheral circulatory system and can even enter bone marrow to create a population of germ cells that are originated from the ovary.
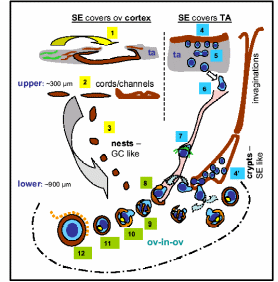
(1) Tunica albuginea stem cells differentiate to OSE, (2) OSE derives chords/channels in upper cortex, (3) fragmented epithelial chord form the granolosa-like epithelial nests. (4) Asymmetric epithelial division produces germ cells (5) which symmetrically divide to increase pool (6) and descend into cortex through cortical vessels (7). Germ cells differentiate to oocytes and picked up by epithelial nests (8) and in steps form the primary follicle (8-12). Germ cells are also delivered through OSE crypts and inclusion cysts (4’). Taken from Bukovsky et al. (2004).
It was assumed that if the germ cells originate from the ovary and are released into the bloodstream it would be possible to replenish the postmenopausal exhaustion of the germ cells by transfusing blood or bone marrow from reproductively active donors and thereby regenerating the ovarian germ cells in the recipient. In rodents this was not the case, rather depleting ovarian epithelial nests became the limiting factor for primary follicle formation, albeit still enough stem cells were generated from OSE stem cells (Bukovsky, 2005). In short, the tunica albuginea mesenchymal cells represent a group of progenitor cells with committed differentiation to two cells types, granulosa and oocytes. The proposed order of transition was (in adults): mesnchyma > epithelium (including granulosa) > germ cell; and for the prenatal stage it was: presumptive epithelium (fetal coelomic) > mesenchyma (perinatal tunica albuginea) > epitheliaum (adult coelomic and granulosa) > germ cell. Thus, OSE somatic stem cells have the potential to divide asymmetrically to produce small daughter “self” cells and larger germ cells. The pattern of division is influenced by two types of microvascular-associated cells, the Monocyte-derived cells (MDC)/ dendritic cell precursor (DCP), and T-cells. The amoeboid germ cells were shown in direct contact with such vascular cells in the OSE and tunica albuginea. The resultant germ cells undergo symmetric meiotic division to produce oocytes. This division takes place while germ cells are migrating downwards into the ovarian cortex before being trapped by epithelial nests. Epithelial-to-granulosa cell transition in fragmented chords seems to be a symmetric division of epithelial stem cells.
The concept of OSE origin of progenitor oocytes was a contradiction to a well-established classical doctrine that is, most mammalian females lose the capacity of germ-cell renewal during fetal life such that a fixed reserve of germ cells (oocytes) enclosed within follicles is endowed at birth (Johnson et al., 2004). This group worked on a concept that treatment of pre-pubertal female mice with a germ cell-mitosis inhibitor busulphan exhausts the reserve of primordial germ cell (PGC) reserve in adulthood. These results indicated that juvenile mouse carry the mitotically active germ cells that continuously replenish the follicle pool. During pre-puberty primordial follicles never develop further to the primary follicle and degenerates to atresis and clears off. In adult though, complete follicle differentiation occurs and after ovulation, signals for the next round of estrous period enables filling up of the next primordial follicle with PGC. The PGC’s are of embryonic origin, they migrate to embryonic gonads and develop into oogonia which multiply mitotically until they are covered with fetal granulosa cells in unorganized chords or sheets and then meiosis derives the oocytes. These presumptive PGC’s later acquire the capabilities of multipotent stem cells and can differentiate into either embryonic germ cells or embryonal carcinoma cells. Post-natal, a fixed number of PGC’s which are destined to produce germ cells are released in the bloodstream and are reserved as a population of stem cells in the bone marrow. Time-to-time these PGC’s are delivered to the ovaries to be later developed into primordial and then to developed follicles or degenerate. About one-third of the PGC’s in the reproductive age of a female degenerate, while the rest release the ova for menstruation or fertilization. As menopause approaches the PGC’s start to deplete and get exhausted (Kerr et al. 2008). From this doctrine, it is clear that PGC’s are targeted from elsewhere in the body to the ovary and here they are deposited in the OSE layer. Johnson et al. (2004) did find the presence of PGC’s in the OSE layer but they confirmed that these cells were not intimately associated with the smaller squamous epithelial cells in any type of structure reminiscent of a follicle (Fig. 9).
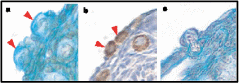
(a) Presumptive germ cells in the OSE but not intimately associated, (b) immuohistochemical staining with germ-cell specific mouse Vasa homologue (MVH) locate germ cells in OSE and in upper cortex, and (c) Germ cells undergoing mitosis across OSE. Taken from Johnson et al. (2004).
Collectively, two views were put forth to explain the origin of germ cells in higher vertebrates – mammals and birds, the oocyte “storage” and “continuous formation” theories. The “storage” doctrine is based o a view that oocyte number is pre-fixed in an embryonic state and never the number increases in adulthood (Johnson et al., 2004). The “continuous formation” theory suggests that the germ cells are produced and degenerated from the cyclical proliferation of the OSE stem cells (Bukovsky et al. 2004). Subsequent research by Bukovsky & Caudle (2008) proposed a new concept harmonizing the previous theories, called “prime reproductive period” theory (Fig. 10). The “storage” theory fits to two periods in human life: from termination of fetal oogenesis to onset of puberty (10-12 years), and following the end of prime reproductive period (> 38 years). At these age groups, the embryonic PGC’s are the source of primordial follicles, and they are subsequently degenerate prior to follicle development and differentiation. The “continued formation” theory is befitting for the length of the prime reproductive period (12-38 years) in which the follicular renewal and availability of fresh oocytes for the next progeny are continuously ensured from the existing OSE layer. It’s only when the OSE layer is disturbed or the ovary is surgically removed in the prime reproductive period, the embryonic PGC’s start to complement the shortage of OSE-derived oocytes. Interestingly, in the reproductive period, cyclic PGC production is related to cyclic gonadotropin release from the pituitary, which means PGC numbers under “continuous formation” appear to be under hormonal control. Conversely, “storage” PGC’s numbers in childhood and post-menopause seems to be not correlated with hormonal concentrations.
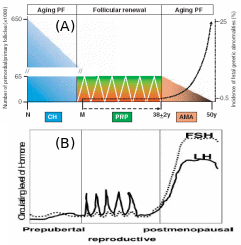
Post-natal atresis of “storage” PGC sharply decrease the number, which cyclically maintains itself during reproductive phase by “continuous production” coinciding with hormonal level, and after menopause hormonal rise brings about genetic aberrations even though the “stored” PGC’s start to deplete. Adapted from Bukovsky & Caudle (2008).
Epidemiology and heredity of ovarian epithelial cancer (OEC)
Numerous papers have projected that ovarian cancer is the fifth leading cause of cancer deaths, and results in the highest mortality rate among gynecological cancers. Over 90% of ovarian cancers arise from surface epithelium. The extent of lethality in this cancer is the highest among various malignancies. At the initial stage, the five-year survival chances are only 45% and as the disease advances, the chances in the patients reduce further to 30% (Ponnusamy & Batra, 2008; Landen et al. 2008). Most ovarian cancers have no racial or ethnic prevalence, even though the incidences are generally higher in Western countries due to industrialization and cultural habits. Though the occurrence of ovarian cancer is recognized as sporadic, about 5-10% of incidences have familial history, and risk among first-degree relatives (mother, sister and daughter) increases by 50% (Murdoch & McDonnel, 2002). Wong & Auersperg (2003) and Sowter & Ashworth (2005) reviewed the nature of inheritable traits responsible for the familial lineage of OEC. In many cases, individuals carrying germline mutations in one of the alleles of the tumor suppressor genes BRCA1 or BRCA2 are at a significantly higher risk of acquiring breast/ovarian cancers (Fig. 11). Women inheriting BRCA1 or BRCA2 genes have ~40% or ~10% risk, respectively, of developing ovarian cancer by the age of 70. To a lesser extent, ovarian cancer can be inherited in a family with cases of heredity non-polyposis colon cancer (HNPCC). In this kind of cancer, the DNA mismatch repair genes, MSH2 and MLH1 are reportedly mutated. The BRCA1 and BRCA2 codes for proteins that are responsible for double stranded DNA breaks by homologous recombination. Consequently, several chromosomal abnormalities and genetic instability lead to the onset of a cancerous transformation of mammary and ovarian epithelia. While BRCA protein is widely expressed in all kind the cells, the prevalence of BRCA mutations in some tissues and not the others relate to the microenvironment of a particular tissue which becomes crucial for pathogenesis. Internalization of OSE to form inclusion cysts is a highly proliferative act of the epithelial cells, which happens cyclically in the ovary and endometrium during the menstrual cycle. The almost same extent of epithelial proliferation takes place in ducts of mammary glands during puberty, pregnancy and lactation. Despite the genotoxic/mitogenic effect of the local environment and inhibition of apoptosis, the epithelial cells sustain the healthy progression so long as the BRCA proteins remain functional. Obviously, in the case of BRCA1/2 mutations, the cells would tend to transform cancerous if mutated from paracrine/autocrine mitogenic action. The BRCA1-associated cancers are more pronounces in the female epithelial cells rather than male ones. Several reasons were ascribed, like 1) mutation affects ovarian and mammary tissues, 2) hormonal factors particularly estrogens have a direct impact on the aggravation of the cancerous transformation of mutated cells, and 3) BRCA1 is found to contribute to X-chromosome inactivation, which is a process specific for female cells, and breast and ovarian carcinoma cells lacking BRCA1 show evidence of defects in X-chromosome structure. Fig. 12 shows the process of follicle ovulation and the consequent events leading to OEC.
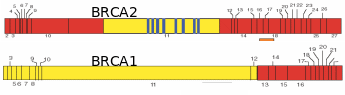
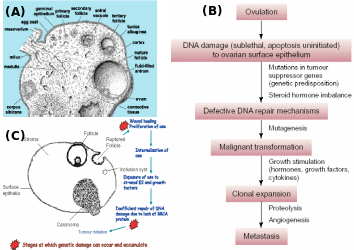
(A) Ovarian architecture showing different stages of follicles, ovulation and CL formation, (B) progressive role of ovulation in chronology of OEC, and (C) OSE during repeated ovulation and mitogen exposure and accumulated mutations particularly in individuals with BRCA lesions invaginates as inclusion cysts and epithelial cells turn malignant and then manifest as serous carcinoma. Adapted from Pan & Huang (2008) and Sowter & Ashworth (2005).
Histopathological evidence for the origin of OEC
Auersperg et al. (2001) proposed that histologically surface epithelial carcinoma cannot be considered in isolation from the other ovarian epithelia derived from the Müllerian duct during embryonic development. As mentioned, in a fetus the primitive form of gonadal coelomic epithelial lining invaginates as Müllerian duct, which eventually derives the epithelia of oviduct, endometrium and uterine cervix. Histologically, the OSE malignancy can be considered to be serous (oviduct/fallopian tube-like), endometrioid (endometrium-type) and mucinous (endocervical-like) adenocarcinomas, with serous adenocarcinoma comprising about 80% share of the total ovarian epithelial carcinoma. Early malignant changes in OSE occur more frequently within the cells of crypts and inclusion cysts that protrude in the cortex region, rather than in the surface monolayer. The cell of origin of ovarian epithelial tumors is a debatable issue. According to Auersperg & Woo (2008), high-grade serous ovarian carcinoma of OSE does not arise from the OSE layer, as was previously thought, but its origins from a distal fimbriated part of the fallopian tube/oviduct, and are only secondarily manifested in metastasis within the ovarian surface. This concept is based on the common embryonic origin of OSE and the Müllerian ducts in the fetal coelomic epithelium. Developmentally, there is a common origin of extra-ovarian peritoneum, the OSE and the oviductal epithelium, originating from coelomic epithelium. The coelomic epithelium-derived epithelia remain contiguous in adults in spite of the wide difference in their structures and functions that signify them as parts of separate organs. Histological analysis reveals that there are no sharp boundaries separating the three otherwise distinctly placed epithelia. The various proliferation indicative markers also show the transition from OSE to the oviductal epithelium and vice versa.
Another school of thought is that OEC arises from the OSE per se that covers the ovarian surface and not from the fimbriated part of the fallopian tube that has Müllerian duct lineage. The two arguments were debated by Dubeau (2008) to arrive at some conclusion on the cell of origin of OEC. A model has been created to rationale the validity of each argument (Fig. 13). In the first line of evidence, none of the surgically removed normal ovarian constituents were found to be lined with epithelial cells resembling those cells lining the fallopian tube, endometrium or endocervix. Almost all benign and malignant ovarian tumors originate from cystic structures. Cysts that are less than 1 cm, referred to as inclusion cysts, are layered with coelomic epithelium. The ovarian cortex also harbors larger than 1 cm cysts of unknown origin with fallopian tube, endometrium and endocervix-like epithelial lining, called metaplastic cysts which differentiate into cancerous tumors. Benign ovarian epithelial tumors can develop even outside the ovary, and in individuals whose ovaries have been surgically removed. Male transgenic mice with embryonic defects in the Müllerian tract develop uterus with attached fallopian tube and cervix but lacks ovaries, suggesting that there is virtually no association between these organs even at the embryonic stage. Further to this, the epithelial cells of serous, endometrioid, and mucinous ovarian carcinoma, as opposed to the coelomic epithelium, express a set of HOX genes suggesting their common Müllerian origin. A possible justification for this ambiguity could be that, apart from organs with possibly common epithelial lining of Müllerian lineage, there exist also secondary Müllerian epithelia associated with the paraovarian region and which has the potential of cancerous growth. The secondary Müllerian system sometimes extends to the ovarian medulla or even in the deeper cortical regions and may account for OEC and many other extraovarian cancers not primarily associated with the Müllerian tract. These findings support a hypothesis of the extra-Müllerian origin of OEC.
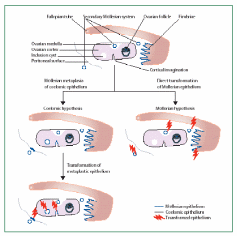
Left panel – Ovarian coelomic epithelial lining of inclusion cysts undergo metaplasia to Müllerian-like epithelium and then undergo malignant transformation. Right panel – Peritoneal and Müllerian epithelium outside ovary directly undergo tumorigenesis and enter through secondary Müllerian structures into ovarian cortex. Taken from Dubeau (2008).
Differential gene expression and physiology of normal and cancerous OSE cells
We have seen a cascade of reactions initiated from u-PA released from normal OSE and culminating in collagenase action of MMP’s cleaving the cadherins and other adhesion connective tissues. The cancerous OSE cells also secrete u-PA and activate latent collagenases digesting the basement membranes and interstitial connective tissue matrix, providing ample space for cancer cells to invaginate (Murdoch & McDonnel, 2002). To get a deeper insight into the trans-membrane signaling associated with OEC, Salini et al. (2007) carried out an analysis of ECM proteins during ovarian cancer progression. Gene expression of 16 ECM proteins including the one called syndecan 1 was monitored in normal OSE and in benign and malignant ovarian serous tumors. Basically, overexpression of syndecan 1, collagen type IV α-2, elastin microfibril interphase located protein 1, and laminin 5 in OSE and underneath tissues were associated with cancerous growth. Dysregulation of ECM has implications in the progression of not only the OEC but also breast, colorectal and pancreatic cancer. Syndecan 1 has been shown to participate in proliferation, migration, and cell-matrix interactions, and is normally found on the surface of many types of cells, including epithelial cells and fibroblasts. This proteoglycan has the ability to interact with fibrous proteins of the ECM, and it can bind and sequester growth factors including fibroblast growth factors and several other localized growth factors. The role of these alterations in OEC has not been clearly established yet though.
Most of the recent investigation on cancer physiology was aimed at comparative gene expression analysis and proteome analysis of normal and malignant tissues. Leung & Choi (2007) described the major neoplastia-associated protein expression in the ovarian epithelium. During neoplastic progression, the typical epithelio-mesenchymal conversion diminishes and the cells become committed to the epithelial characteristics like an expression of E-cadherin, the receptor of hepatocyte growth factor (c-met), and secretory products like mucins. Besides the BRCA1/2 mutations, alterations are also seen in several tumor-suppressor genes viz. p53, PTEN, Lot-1, OVCA-1, DOC-2 and NOEY2. A tight interaction of cyclins/cyclin-dependent kinases (CDK) and CDK inhibitors (CDKI) like p21 and p27 that regulate normal growth gets perturbed. Unlike the normal OSE cells, cancer cells have a high level of telomerase, which maintains telomere length and resultantly evades apoptosis. Several oncogenic signaling molecules like phosphoinositide 3-kinase (P13K)/Akt, MAPK, tyrosine kinase receptors and epidermal growth factor receptors (EGFR) are also up-regulated. The mitogen-activated protein kinases (MAPK) respond to external stimuli like inflammatory and mitogenic substances through cell surface tyrosine kinase- and G protein-coupled receptors, and bring about a cascade of reactions that transcriptionally activate a set of proteins that regulate cell growth, division and differentiation by a diverse mechanism. These signal transduction pathways are ultimately governed by the peptidal hormones, GnRH, gonadotropins, activin, inhibin and some steroidal hormones.
One of the first analyses of differentially expressed genes was carried out in the cell lines derived from normal human OSE (HOSE) and Cedars-Sinai Ovarian Cancer (CSOC) cells (Ismail et al. 2000). For this, total RNA from both the cell lines were used for cDNA synthesis. After subtractive hybridization, a total of 255 nonredundant genes were identified by filter array hybridization with designated primers. Genes preferentially expressed in CSOC were of special interest and they were identified as collagen type XV and VI α 1, collagen type I α 2, osteoblast-specific factor 2, annexins etc, based on the BLAST search results. Though a conclusive physiological relevance of such gene expression could not be elucidated, almost all the gene homologue-derived proteins were associated with cellular surface properties and adhesion. In another similar investigation, Matei et al. (2002) applied cDNA microarray analysis of the normal and cancerous epithelial cells and the expression analysis was carried out using specific primers. The PCR products were sequenced for a BLAST search of gene homologues. A much better picture of cancer-specific gene expression could be obtained by this technique, and identified genes were of antilukoprotease, aortic carboxypeptidase–like protein, collagen type XI α 1, osteoblast-specific factor 2, integrin, membrane-type MMP, heat shock protein 27 and many others. Although how this attribute facilitates oncogenesis was unexplained, these studies provided several ovarian cancer-specific markers for prognosis of disease much before uncontrollable metastasis takes place.
The application of new genomic technologies such as comparative genome hybridization and microarray expression profiling has helped elucidate many of the important genetic events that may lead to ovarian cancer (Landen et al. 2008). Pathological analysis revealed two grades of serous carcinomas, low- and high malignant grades. The complete physiological events and the nature of proteins involved are presented in Fig. 14. Among the over-expressed or under-expressed genes were: 1) cell growth promoters like EGFR (HER1/2) – membrane TK receptors that promote cell growth; K-RAS – G-protein that promotes growth through MAP kinase pathway; BRAF – promotes growth through MAP kinase pathway; 2) rendering insensitivity to antigrowth signals like TGF- β-Ligand inhibits growth through retinoblastoma (Rb) activation; C-MYC – transcription factor and cell cycle mediator; Cyclin D/CDK4/6 and E/CDK2 – advancing from G1 to S phase; Cyclin B/CDK1 – advancing cell cycle into M phase, NFκB – transcription factor and effector of many survival pathways, 3) inhibition of apoptosis like PIP3/AKT – AKT (activated by PIP3) mediated apoptosis inhibition; p53 – promotes cell cycle arrest/apoptosis with DNA damage, BRCA1/2 – cofactor for transcription factors and caretaker of genome; 4) enhanced angiogenesis like VEGF/VEGFR – Ligand/receptor complex that induce angiogenesis, and 5) promotion of invasion and metastasis like MMPs – degrade extracellular matrix; FAK – cofactor TK that promote adhesion, proliferation and survival, and E-cadherin – promotes adhesion. A model of ovarian carcinogenesis explained that upon mitogen induction, OSE cells undergo K-RAS/BRAF mediated cell growth for low-grade carcinoma, whereas if EGFR/HER2 signal transduction is triggered, the high-grade carcinoma would result. Likewise, there is a difference in apoptosis inhibition signals distinctly promoting the two grades of cancers. Beyond this point, the inductive role of the remaining factors is equal in magnitude for both grades of malignancies.
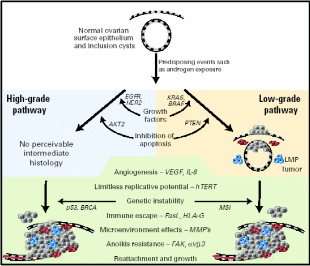
Low/high grade serous and other ovarian malignant transformation includes unregulated growth, resistance to antigrowth signals, inhibition of apoptosis, evasion of immune recognition, limitless replication, induction of angiogenesis, and invasion of basement membrane with designated signal pathways and proteins. Cells colored red are MMP-producing and blue are inflammatory. Taken from Landen et al. (2008).
Another interesting approach in this direction has been to prepare a microarray of differential splicing patterns of normal and cancerous OSE cultured cells, which either knock out the expressed protein or render them functionally altered. In both situations, the OSE cells approach tumorigenesis (Klinck et al. 2008). The splicing variation arises from mutations in tumor suppressor genes at the splicing sites. A number of aforementioned cancer-associated genes controlling cell growth, adhesion, invasion and angiogenesis are mutated such that splicing profile changes can be correlated to the cancer progression. In this study, typically two genes were short-listed whose splicing was maximally affected, and they had high fecundity with ovarian cancer. These were the multi-drug resistant proteins ABCC1 and p53 regulator MDM2.
A comparative proteome profile of normal and cancerous OSE cell lines was examined by Gagne et al. (2007) by applying isobaric tags for relative and absolute quantification (iTRAQ) and 2-D electrophoresis coupled to LC MS/MS and by applying BLAST search to get homolog sequences. Some of the keys differentially expressed proteins were: Insulin-like growth factor 2 mRNA-binding protein 1, Collagen alpha-1(III) chain precursor, Aldehyde dehydrogenase 1A1, Heat shock 70 kDa protein, Proliferation-associated protein 2G4, Heterogeneous nuclear ribonucleoprotein L, Thioredoxin-like protein 2, Regulator of chromosome condensation, Eukaryotic translation initiation factor 4B, Keratin type I/II cytoskeletal 19/8 etc. The most profound difference was found for the proteins associated with locomotion and localization, cell organization and biogenesis, cell motility, cytoskeleton organization, actin-filament processing, angiogenesis, mRNA metabolism, splicing and peptidal proline metabolism.
Cancer stem cells constitute a small proportion of OSE stem cells
Increasing evidence supports the hypothesis that OSE tumor growth capacity depends on cancer stem cells (CSC’s) that constitute a small proportion of OSE stem cells (Pan & Huang, 2008). CSC’s are responsible for the aggressiveness of the disease, metastasis and resistance to therapy. In OEC cells a small subset of tumor stem cells, called side population (SP), actually proliferates as CSC’s and the rest of the population behaves like stem cells destined for damaged tissue repair. SP can be detected by its ability to efflux the DNA-binding Hoechst 33342 dye through the ABC membrane transporter. There are a variety of cell surface protein markers, named CD’s, assigned to CSC’s of different lineage, for e.g. mammary CSC– CD44 + CD24, colon CSC– CD133, and ovarian CSC– CD133 + CD117. Interestingly, CD-133 is a central nervous system stem cell marker also expressed in ovarian CSC. Ponnusamy & Batra (2008) projected three properties of CSC, 1) only a minority of cancer cells within the tumor cell population is endowed with tumorigenic potential, 2) tumorigenic cancer cells have distinct cell surface markers from others, and 3) tumors grown from tumorigenic cells ultimately contain a mixed population of both tumorigenic and non-tumorigenic cells, thus cells maintain phenotypic heterogeneity in tumor formation. Like other stem cells, the CSC’s have the ability to self-renew, generate more CSC’s, and some of them differentiate to putative malignant cells while others remain undifferentiated. One pertaining question is how the multipotent stem cells decide whether to renew to identical cells or to differentiate into new phenotypes. Recently, various signaling pathways were discovered associated with this mechanistic change of stem cells. Among them are the Sonic Hedgehog (Shh) and Notch and Wnt signal transduction pathways whose relationship with the fate of stem cells is shown in Fig. 15. While these pathways are operative for the self-renewal of normal OSE stem cells, they also function for the CSC self-renewal. It is only when the cells are mutagenized the signaling pathway alters such that the same cells begin to differentiate into the tumor mass. The dual mechanistic property of CSC’s is not just an in vivo property but can also be demonstrated in the cell’s cultures. While these differentiating and aggressively tumorizing CSC’s initially respond to the therapeutic drugs, later they develop resistance phenotype by a further round of differentiation into drug-resistant cells. In one such assay using mouse CSC’s, it was found that initially, these tumorigenic cells responded well to the chemotherapeutic drugs mitoxantrone and daunorubicin, but eventually, they expressed multi-drug-resistant gene 1 and breast cancer resistance protein 1 (BCRP1), and such differentiated CSC’s became drug-resistant (Szotek et al. 2006).
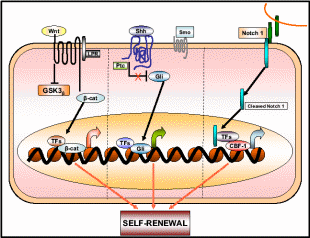
Wnt, Shh, and Notch 1 pathways contribute to the self-renewal of stem/progenitor cells. Once deregulated or mutated these pathways may lead to oncogenesis. Taken from Ponnusamy & Batra (2008).
CSC tumorigenesis whether a genetic or an epigenetic phenomenon?
The concept of epigenetic progenitor origin of human cancer was put forth by Feinberg, Ohlsson & Henikoff (2006). These include global or gene-specific alterations in DNA, which manifest through hypo- or hypermethylation or chromatin (histone) hypoacetylation. Silencing of several aforementioned tumor suppressor genes is associated with hypermethylation of the promoter region and hypoacetylation within the corresponding chromatin region. Moreover, these epigenetic factors also activate oncogene expression. Most likely these epigenetic changes distinguish between normal and cancerous stem cells. Dominantly acting oncogenes and recessively acting tumor-suppressor genes may account for the onset of cancer. The three points of control of multi-potent normal stem cells to carcinogenic primary cancer cells and further differentiation to more complicated attributes are presented in an epigenetic progenitor model presented in Fig. 16.
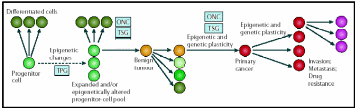
Cancer arises in three steps: (a) aberrant regulation of tumor-progenitor genes (TPG) due stem cell themselves or the external factors, (b) mutation in tumor suppressor genes (TSG) or rearrangement of oncogenes (ONC) which increases risks of tumors but do not by themselves initiate tumors, (c) further sequential epigenetic plasticity increases tumor evolution (variations) ultimately results in invasion, metastasis and drug resistance. Taken from Feinberg, Ohlsson & Henikoff (2006).
Zang et al. (2008) proposed that epigenetic factors also regulate micro(mi)RNA expression, which in turn can silence ovarian oncogenes. Evidence shows that deregulation of miRNA expression is related to ovarian cancer. miRNA are generally expressed within the non-coding region of chromatin. Differential expression of miRNA was profiled in cancerous and normal OSE cell cultures. Overall, there are miRNA’s, called mir-15a, mir-34a and mir-34b, was found to be prominently down-regulated in cancerous relative to normal OSE cells. Since miRNA selectively controls the stability of mRNA by cleaving it, it was expected that lowering of miRNA’s level would selectively up-regulate several cancer-associated mRNA’s. Most deletions within specific regions of chromosomes #14, 19 and X contribute to miRNA down-regulation, but about one-third of miRNA’s are also regulated by epigenetic means. For example, mir-34b is down-regulated by tumor-suppressor p53, though it is also down-regulated by epigenetic factors in OEC. miRNA profiling of OSE has recently gained a lot of importance because it is possible to use them as blood-based markers for the early detection of OEC. Wyman et al., (2009) prepared a differential miRNA’s cDNA libraries for human OSE and found a completely new family of miRNA’s (miR-200 family) which expressed at a low level in HOSE but substantially high in ovarian cancer. miRNA belonging to this family has an important role to play in repressing the mRNA’s of E-cadherin transcriptional repressor proteins ZEB1 and ZEB2, whose function is to promote the epithelial-to-mesenchymal transition.
Aims of the project
The OSE plays an important role in normal ovarian physiology, specifically in the cyclic ovulatory ruptures and repair. Thus, the mitogenic activity of OSE cells in the reparative process may favour the survival of OSE with accumulated mutations leading to OSE cell transformation and malignancy.
The overall aim of this thesis is to define how the physiological reproductive events affect the ovarian surface epithelium activity specifically:
- To examine the proliferative activity of the OSE through the regular ovarian cycle and pregnancy by monitoring proliferation in vivo using immunohistochemistry (IHC) for cell cycle markers (PCNA and Ki-67) (sheep and cow) and BrdU incorporation (marmoset).
- Using primary culture system of OSE (sheep and cow) to examine the effect of steroids and gonadotropin on OSE proliferation and apoptotic cell death.
- Study the expression and localization of P53 tumour suppressor gene within OSE cells in consideration of its role as a genomic guardian gene (cows).
Different species were used as models to examine the physiological differences among OSE from different animals.
References
- Ahmed, N., Maines-Bandiera, S., Quinn, M.A., Unger, W.G., Dedhar, S. & Auersperg, N.(2006). Molecular pathways regulating EGF-induced epithelio-mesenchymal transition in human ovarian surface epithelium. American Journal of Physiology- Cell Physiology, 290, 1532-1542.
- Auersperg, N., Wong, A.S.T., Choi, K.C., KANG, S.K. & Leung, P.C.K.(2001). Ovarian Surface Epithelium: Biology, Endocrinology, and Pathology. Endocrine Reviews, 22(2), 255-288.
- Auersperg, N & Woo, M.M.M.(2008). The origin of ovarian carcinomas: A developmental view. Gynecologic Oncology, 110, 452–454.
- Bukovsky, A.(2005). Can ovarian infertility be treated with bone marrow- or ovary-derived germ cells? Reproductive Biology and Endocrinology, 3:36 doi:10.1186/1477-7827-3-36.
- Bukovsky, A., Caudle, M.R., Svetlikova, M. & Upadhyaya, N.B.(2004). Origin of germ cells and formation of new primary follicles in adult human ovaries. Reproductive Biology and Endocrinology, 2:20, doi:10.1186/1477-7827-2-20. Web.
- Bukovsky, A. & Caudle, M.R.(2008). Immune Physiology of the Mammalian Ovary – A Review. American Journal of Reproductive Immunology, 59, 12–26.
- Curry, T.E. & Osteen, K.G.(2003). The Matrix Metalloproteinase System: Changes, Regulation, and Impact throughout the Ovarian and Uterine Reproductive Cycle. Endocrine Reviews, 24(4), 428–465.
- Dubeau, L.(2008). The cell of origin of ovarian epithelial tumours. Lancet Oncology, 9, 1191–1197.
- Duke, K.K.(1978). Nonfollicular Ovarian Components. In Jones, R.E. (Ed.), The vertebrate Ovary-Comparative biology and Evolution (pp. 563-582). New York: Plenum Press.
- Feinberg, A.P., Ohlsson, R. & Henikoff, S.(2006). The epigenetic progenitor origin of human cancer. Nature Reviews, 7, 21-33.
- Gagne, J-P., Éthier, C., Gagne, P., Mercier, G., Bonicalzi, M-E., Mes-Masson, A-M., Droit, A., Winstall, E., Isabelle, M. & Poirier, G.G.(2007). Proteome Science. 5:16 doi:10.1186/1477-5956-5-16. Web.
- Ismail, R.S., Baldwin, R.L., Fang, J., Browning, D., Karlan, B.Y., Gasson, J.C. & Chang, D.D.(2000). Differential Gene Expression between Normal and Tumor-derived Ovarian Epithelial Cells. Cancer Research, 60, 6744–6749.
- Johnson, J., Canning, J., Kaneko, T., Pru, J.K. & Tilly, J.L.(2004). Germline stem cells and follicular renewal in the postnatal mammalian ovary. Nature, 428, 145-150.
- Kerr, C.L., Hill, C.M., Blumenthal, P.D. & Gearhart, J.D.(2008). Expression of pluripotent stem cell markers in the human fetal ovary. Human Reproduction, 23, 589-599.
- Klinck, R., Bramard, A., Inkel, L., Dufresne-Martin, G., Gervais-Bird, J., Madden, R., Paquet, E.R., Koh, C., Venables, J.P., Prinos, P., Jilaveanu-Pelmus, M., Wellinger, R., Rancourt, C., Chabot, B. & Elela, S.A.(2008). Multiple Alternative Splicing Markers for Ovarian Cancer. Cancer Research, 68(3), 657-663.
- Landen Jr, C.N., Birrer, M.J. & Sood, A.K.(2008). Early Events in the Pathogenesis of Epithelial Ovarian Cancer. Journal of Clinical Ontology, 26(6), 995-1005.
- Lee, J.M., Dedhar, S., Kalluri, R. & Thompson, E.W.(2006). The epithelial–mesenchymal transition: new insights in signaling, development, and disease. The Journal of Cell Biology, 172(7), 973–981.
- Leung, P.C.K. & Choi, J-H.(2007). Endocrine signaling in ovarian surface epithelium and cancer. Human Reproduction Update, 13(2), 143–162.
- Matei, D., Graeber, T.G., Baldwin, R.L., Karlan, B.Y., Rao, J. & Chang, D.D.(2002). Gene expression in epithelial ovarian carcinoma. Oncogene, 21, 6289 – 6298.
- Morrison, S.J. & Kimble, J.(2006). Asymmetric and symmetric stem-cell divisions in development and cancer. Nature, 441, 1068-1074.
- Murdoch, W.J. & McDonnel, A.C.(2002). Roles of the ovarian surface epithelium in ovulation and carcinogenesis. Reproduction, 123, 743–750.
- Pan, Y. & Huang, X.(2008). Epithelial Ovarian Cancer Stem Cells—A Review. International Journal of Clinical and Experimental Medicine, 1, 260-266.
- Parenti, L.R. & Greier, H.J.(2004). Evolution and Phylogeny of Gonad Morphology in Bony Fishes. Integrative and Comparative Biology, 44, 333–348.
- Parrott, J.A., Kim, G. & Skinner, M.K.(2000). Expression and Action of Kit Ligand/Stem Cell Factor in Normal Human and Bovine Ovarian Surface Epithelium and Ovarian Cancer. Biology of Reproduction, 62, 1600–1609.
- Pieau, C. & Dorizzi, M.(2004). Oestrogens and temperature-dependent sex determination in reptiles: all is in the gonads. Journal of Endocrinology, 181, 367–377.
- Ponnusamy, M.P. & Batra, S.K.(2008). Ovarian cancer: emerging concept on cancer stem cells. Journal of Ovarian Research, 1:4, doi:10.1186/1757-2215-1-4.
- Ravaglia, M.A. & Maggese, M.C.(2002). Oogenesis in the swamp eel Synbranchus marmoratus (Bloch, 1795) (Teleostei; synbranchidae). Ovarian anatomy, stages of oocyte development and micropyle structure. BIOCELL, 26(3), 325-337.
- Salani, R. Neuberger, I., Kurman, R.J., Bristow, R.E., Chang, H-W., Wang, T-L. & Shih, I-M.(2007). Expression of Extracellular Matrix Proteins in Ovarian Serous Tumors. International Journal of Gynecological Pathology, 26, 141–146,
- Sowter, H.M. & Ashworth, A.(2005). BRCA1 and BRCA2 as ovarian cancer susceptibility genes. Carcinogenesis, 26(10), 1651-1656.
- Sretarugsa, P., Weerachatyanukula, W., Chavadeja, J., Kruatrachueb, M. & Sobhona, P.(2001). Classification of Developing Oocytes, Ovarian Development and Seasonal Variation in Rana tigerina. ScienceAsia, 27, 1-14.
- Szotek, P.P., Chang, H.L., Brennand, K., Fujino, A., Pieretti-Vanmarcke, R., Celso, C.L., Dombkowski, D., Preffer, F., Cohen, K.S., Teixeira, J. & Donahoe, P.K.(2008). Normal ovarian surface epithelial label-retaining cells exhibit stem/progenitor cell characteristics. Proceedings in National Academy of Science U.S.A., 105(34), 12469-12473.
- Szotek, PP., Pieretti-Vanmarcke, R., Masiakos, P.T., Dinulescu, D.M., Connolly, D., Foster, R., Dombkowski, D., Preffer, F., MacLaughlin, D.T. & Donahoe, P.K.(2006). Ovarian cancer side population defines cells with stem cell-like characteristics and Mullerian Inhibiting Substance responsiveness. Proceedings in National Academy of Science U.S.A., 103(30), 11154–11159.
- Wong, A.S.T. & Auersperg, N.(2003). Ovarian surface epithelium: family history and early events in ovarian cancer. Reproductive Biology and Endocrinology, 1:70, doi:10.1186/1477-7627-1-70.
- Wyman, S.K., Parkin, R.K., Mitchell, P.S., Fritz, B.R., O’Briant, K., Godwin, A.K., Urban, N., Drescher, C.W., Knudsen, B.S. & Tewari, M.(2009). Repertoire of microRNAs in Epithelial Ovarian Cancer as Determined by Next Generation Sequencing of Small RNA cDNA Libraries. PLoS ONE, 4(4), e5311, doi:10.1371/journal.pone.0005311.
- Zhang, L., Volinia, S., Bonome, T., Calin, G.A., Greshock, G., Yang, N., Liu, C.G., Giannakakis, A., Alexiou, P., Hasegawa, K., Johnstone, C.N., Megraw, M.S., Adams, S., Lassus, H., Huang, J., Kaur, S., Liang, S., Sethupathy, P., Leminen, A., Simossis, V.A., Sandaltzopoulos, R., Naomoto, Y., Katsaros, D., Gimotty, P.A., DeMichele, A., Huang, Q., Buetzow, R., Rustgi, A.K., Weber, B.L., Birrer, M.J., Hatzigeorgiou, A.G. Croce, C.M. & Coukos, G.(2008). Genomic and epigenetic alterations deregulate microRNA expression in human epithelial ovarian cancer. Proceedings in National Academy of Sciences U.S.A., 105(19), 7004–7009.