A General Description
Laser systems are widely used in engineering and industry; lasers are essential for targeted monochromatic irradiation with a coherent beam. There are many different types of lasers, depending on the energy pumping mechanism, the type of source used and the corresponding wavelength of the monochromatic beam. One of these options are tunable lasers, systems whose wavelength of emission can be adjusted to a spectral range depending on the specific application.
For tunable lasers it is true that the width of the spectral range within which the laser wavelength can be changed is much wider than the width of the spectral line. The basic process for producing laser radiation is the pumping process, which is realized by transferring energy from an external source, such as a crystal, into the amplifying medium of the laser. Thus, most tunable lasers are based on solid-state sources pumped by lamps filled with noble gases. When a voltage is applied, the noble gas is excited and the electrons of the molecule move to upper orbital levels, causing the source to be excited.
For example, the BeAl204:Cr3+ Alexandrite Tunable Laser (ATL) uses an Xe-filled polished single-pulse lamp with a flash duration of up to 100 ms (Walling et al., 1979). The excited electrons in the noble gas collide with the BeAl204:Cr3+, resulting in the excitation of the crystal already, expressed as electromagnetic radiation emitted from the chamber. The laser beam enters a cavity called an optical cavity or amplifying medium, surrounded as a rule by mirrors. The main purpose of using an amplifying medium is to generate standing waves. Some of the optical cavity designs are shown in Figure 1 and Figure 2, which result in the output of a focused laser beam of tunable wavelength.
In a series of tunable lasers, there are a fair number of variations to change the output wavelength: Figure 1 shows just one of these variations. In this solid-state setup, the laser beam hits a diffraction grating after passing through a lens; the controlled tilt of this grating allows the wavelength of the output radiation to be influenced. Figure 2 extends this scheme by proposing the following scheme: the laser beam hits the polymer amplifying medium in the form of a diffraction grating, and then only a certain wavelength is reflected. As in the previous example, the diffraction grating is movable, allowing the output characteristics of the radiation to be adjusted.
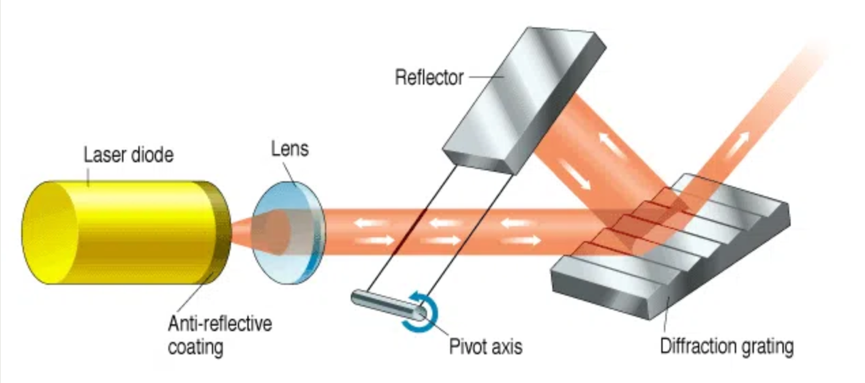
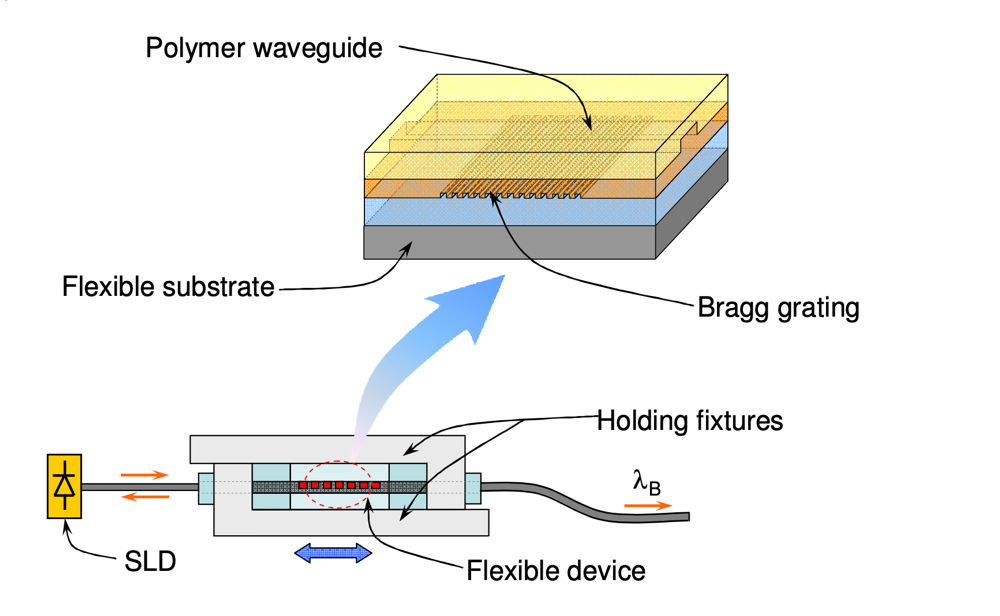
Characteristics of the ATL Facility
For ATL, the standard size of the laser cavity is about 40 cm, surrounded by two mirrors; the total reflection yield is up to 95%. Directly laser rods as solid state sources are about 10 cm long, and 2.5 cm in diameter, and the wavelength range is 701 nm to 794 nm (Walling et al., 1979). The lifetime for a single-pulse ATL after excitation is approximately 260 µsec, which is indicated as sufficient to achieve high-energy radiation on the order of 150 watts. BeAl204 crystal, the carrier of the chromium ions, has high thermal conductivity (up to 0.23 Wcm-1K-1) and mechanical strength, making it possible to use it at moderate plant powers.
Application
The main advantage of using tunable lasers is the possibility of adjusting the wavelength of the output laser light in a controlled manner. The fine wavelength tuning capabilities are widely used in astronomy and laboratory-clinical research, which require optical devices. For example, in the case of astronomical research, ATL can be used to calibrate telescopes as well as control and measurement laboratories.
It is noteworthy that alexandrite lasers, including ATL, are widely used in cosmetology as well. The main property that allows the use of ATL for clinical purposes is realized through the good absorption of laser radiation by collagen, melanin, protein – as a consequence, proteins can radiate with minimal damage to the skin. For example, in laser hair removal procedures, the precise tuning of the radiation output frequencies allows adjusting the epilator’s power.
At the same time, ATL can be used in photodynamic therapy for the treatment of cancer. In particular, during photodynamic therapy photosensitizers — substances that actively respond to electromagnetic excitation — are injected into the body (Ahmetali, 2021). There is an individual wavelength for each photosensitizer to affect the substance. Instead of using different lasers, the use of ATLs allows significant savings in resources and time.
A similar technique can be used for nanoscopic analysis in materials science, where ATL provides information on the characteristics of semiconductors and metals, including gold. Similar directions in the use of ATL allow for estimating the external quantum efficiency of devices and calibrating them if necessary. In addition, ATLs can also be used for military purposes. For military lasers, the idea of which is to territorialize, transmit signals and destroy, fine tuning the output wavelength allows to adjust the operation of the laser system.
Pricing
When discussing the costs of specific ATL installations, it has to be kept in mind that tunable lasers are generally more expensive than classical lasers where the light source is a ruby crystal, for example. However, the benefit of using tunable lasers can be appreciated in the long term: The ability to fine-tune the wavelength of the output radiation saves on the number of instruments used. Instead of several laser units focusing on different radiation frequencies at once, ATLs, like all tunable lasers, allow you to combine them in a single device. Below is a table comparing prices and manufacturers of alexandrite lasers used for various purposes.
Table 1: Price and manufacturer comparison of various tunable alexandrite-based devices
References
Ahmetali, E., Sen, P., Süer, N. C., Nyokong, T., Eren, T., & Şener, M. K. (2021). Photodynamic therapy activities of phthalocyanine-based macromolecular photosensitizers on MCF-7 breast cancer cells.Journal of Macromolecular Science, Part A, 58(11), 748-757. Web.
Bruce, E. (2020). Tunable lasers. IEEE. Web.
Kim, K. J., Kim, J. W., Oh, M. C., Noh, Y. O., & Lee, H. J. (2010). Flexible polymer waveguide tunable lasers. Optics Express, 18(8), 8392-8399. Web.
Walling, J. C., Jenssen, H. P., Morris, R. C., O’Dell, E. W., & Peterson, O. G. (1979). Tunable-laser performance in BeAl 2 O 4: Cr 3+. Optics Letters, 4(6), 182-183.